Guidance on the use of Enterococci as an Indicator in Canadian Drinking Water Supplies
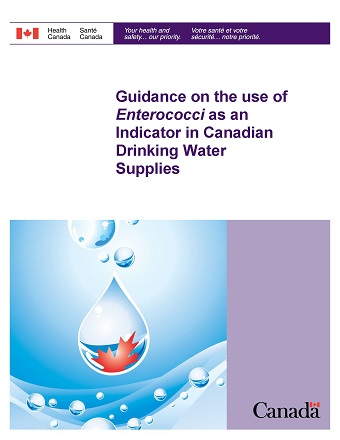
Download the alternative format
(PDF format, 567 KB, 32 pages)
Organization: Health Canada
Date published: 2020-06-26
Health Canada
Ottawa, Ontario
June 2020
This document may be cited as follows:
Health Canada (2020). Guidance on the Use of Enterococci as an Indicator in Canadian Drinking Water Supplies. Water and Air Quality Bureau, Healthy Environments and Consumer Safety Branch, Health Canada, Ottawa, Ontario. (Catalogue No. H144-68/2020E-PDF).
The document was prepared in collaboration with the Federal-Provincial-Territorial Committee on Drinking Water of the Federal-Provincial-Territorial Committee on Health and the Environment.
Any questions or comments on this document may be directed to:
Water and Air Quality Bureau
Healthy Environments and Consumer Safety Branch
Health Canada
269 Laurier Avenue West, Address Locator 4903D
Ottawa, Ontario
Canada K1A 0K9
Tel.: 1-833-223-1014 (toll free)
Fax: 613-952-2574
E-mail: hc.water-eau.sc@canada.ca
Other Guideline Technical Documents for the Guidelines for Canadian Drinking Water Quality can be found on the following web page: www.canada.ca/en/health-canada/services/environmental-workplace-health/reports-publications/water-quality.html
Background on guidance documents
Health Canada works with the provinces, territories and federal agencies to establish the Guidelines for Canadian Drinking Water Quality. Over the years, new methodologies and approaches have led Health Canada, in collaboration with the Federal-Provincial-Territorial Committee on Drinking Water, to develop a new type of document, guidance documents, to provide advice and guidance on issues related to drinking water quality for parameters that do not require a formal guideline under the Guidelines for Canadian Drinking Water Quality.
There are two instances in which the Federal-Provincial-Territorial Committee on Drinking Water may choose to develop a guidance document. The first would be to provide operational or management guidance related to specific drinking water–related issues (e.g., boil water advisories), in which case the document would provide only limited scientific information or health risk assessment. The second instance would be to make health risk assessment information available when a guideline is not deemed necessary.
Guidelines are established under the Guidelines for Canadian Drinking Water Quality specifically for contaminants that meet all of the following criteria:
- exposure to the contaminant could lead to adverse health effects;
- the contaminant is frequently detected, or could be expected to be found, in a large number of drinking water supplies throughout Canada; and
- the contaminant is detected, or could be expected to be detected, at a level that is of possible health significance.
If a contaminant of interest does not meet all these criteria, Health Canada, in collaboration with the Federal-Provincial-Territorial Committee on Drinking Water, may choose not to establish a numerical guideline or develop a guideline technical document. In such a case, a guidance document may be developed.
Guidance documents undergo a similar process as guideline technical documents, including public consultations through the Health Canada website. They are offered as information for drinking water authorities and, in some cases, to provide guidance in spill or other emergency situations.
Executive summary
Enterococci are a bacteriological indicator of fecal contamination. Their presence in drinking water indicates that fecal pathogens may be present which can pose a health risk to consumers. They can be included in a drinking water monitoring program to provide information on the quality of the source water, the adequacy of treatment and the delivery of safe drinking water to the consumer.
Health Canada completed its review of enterococci in drinking water. This guidance document was prepared in collaboration with the Federal-Provincial-Territorial Committee on Drinking Water. It describes the significance, sampling and treatment considerations for the use of enterococci as a bacteriological indicator in the context of drinking water quality and safety.
Assessment
Under a source-to-tap approach to providing high quality drinking water, enterococci are a complementary indicator of fecal contamination. For jurisdictions considering monitoring in addition to the regulatory requirements, this parameter can supplement existing E. coli and total coliforms monitoring programs, to afford a better understanding of microbiological water quality and inform decision-making. An important advantage of the enterococci group is that they are somewhat more resistant to environmental stresses and drinking water disinfectants than E. coli, although both are readily inactivated by drinking water disinfection. Enterococci may persist longer than E. coli in some water environments. They are therefore useful as a bacterial indicator to provide additional insight into fecal contamination issues in systems suspected of being susceptible to fecal contamination— such as undisinfected groundwater sources and distribution systems—but where E. coli has not been found or is infrequently detected. The intent of this document is to provide stakeholders, such as provincial and territorial regulatory authorities, decision makers, water system owners, laboratories and consultants with guidance on how enterococci can be used in a drinking water monitoring program with the objectives of identifying and minimizing microbiological risks in Canadian water systems.
International considerations
Drinking water quality guidelines, standards and/or guidance established by foreign governments or international agencies may vary due to the science available at the time of assessment, as well as the utilization of different policies and approaches. Enterococci are widely used for assessing water quality in many parts of the world, but are not used as frequently as other indicators such as E. coli. The World Health Organization and the Australian drinking water authority indicate enterococci can be used to assess source, treated and distributed water quality, but have not established drinking water guideline values. The European Union’s Drinking Water Directive includes enterococci as an audit parameter for monitoring in drinking water distribution systems with a standard of zero enterococci per 100 mL of water and testing requirements that are less frequent than routine monitoring parameters.
The United States Environmental Protection Agency (U.S. EPA) has not established a drinking water guideline value for enterococci. The Ground Water Rule includes enterococci alongside E. coli and coliphages as options when testing for indicators of fecal contamination when total coliforms are detected in untreated ground water systems.
Table of Contents
- Part A. Guidance on the use of Enterococci as an indicator in Canadian Drinking Water Supplies
- Part B. Supporting information
- B.1 Description, sources, and survival
- B.2 Significance in water
- B.3 Analytical methods
- B.4 Treatment considerations
- B.5 International considerations
- B.6 Research gaps
- Part C. References and acronyms
Part A. Guidance on the use of Enterococci as an indicator in Canadian Drinking Water Supplies
A.1 Goal
The intent of this document is to provide provinces, territories, other government departments and stakeholders (such as water system owners and laboratories) with guidance on how the enterococci group of organisms can be used as an indicator in drinking water monitoring programs.
A.2 Background
In drinking water monitoring programs, indicator organisms are used to assess the microbiological safety of water as the direct detection of pathogenic organisms remains impractical. Globally, E. coli is widely utilized as the first indicator of choice for detecting fecal contamination in drinking water. Using E. coli as the primary indicator of fecal contamination along with total coliforms as a general indicator of microbial water quality contamination, is part of an internationally accepted approach for verifying that drinking water has been adequately treated. However, E. coli is more sensitive to environmental stresses and drinking water disinfectants than many types of bacteria, viruses and protozoa; and therefore the absence of E. coli does not necessarily indicate the absence of these microorganisms. Thus, the use of alternative or additional indicators has been explored by the drinking water industry to provide additional information on water quality. The enterococci group of organisms is one of these indicators.
A.3 Using enterococci in assessing drinking water quality
Enterococci have been used as fecal indicator bacteria for assessing the quality of recreational water, drinking water and water re-use applications in many parts of the world. They are a hardy group of bacteria that provide a strong indication of fecal contamination and are somewhat more resistant to environmental stresses and drinking water disinfectants than E. coli.
Enterococci, like E. coli can be used in drinking water monitoring programs to provide additional information on:
- the quality of the drinking water source;
- the adequacy of drinking water treatment; and
- the microbial condition of the distribution system.
Some groundwater and distribution system monitoring programs in North America and internationally have shown that enterococci are useful as a supplemental indicator, providing better insight into fecal contamination events in vulnerable systems. Given their greater persistence, enterococci may be detected in cases where E. coli is not. Therefore, enterococci can be considered for microbial indicator monitoring in drinking water systems that are suspected of being susceptible to fecal contamination but where E. coli has not been found or has been detected very infrequently.
Enterococci testing may be useful in the following scenarios:
- monitoring programs for groundwater sources that are vulnerable to fecal contamination (e.g., as determined by source water assessments, historical microbiological data, or for systems not meeting the minimum 4 log goal for the removal and/or inactivation of enteric viruses).
- monitoring programs following water mains disinfection procedures for water utilities frequently experiencing disruptions in their drinking water distribution system (e.g., repeated mains breaks or pressure losses).
For other areas such as surface water sources and drinking water immediately post-treatment or leaving the treatment plant, the data is less clear about significant differences in the survival and detection of enterococci and E. coli.
Water utilities considering the use of enterococci need to be aware of the limitations of this group of indicators, including their detection in more non-fecal habitats than E. coli; and the very limited comparative detection data. Until more data are available, it is recommended that enterococci be viewed as a complementary indicator of fecal contamination. Combining multiple indicators affords a better understanding of changes in microbial water quality, and increases the likelihood of detecting periods of higher risk to human health; this is an approach supported by the drinking water literature.
Decisions regarding including enterococci as a monitoring parameter in a regulatory or legislative context should be made by the responsible drinking water authority in cooperation with drinking water utilities. Individual water utilities considering conducting voluntary monitoring for enterococci should base their decisions on a system-specific assessment. Utilities should also consult with the responsible drinking water authority to confirm system-specific requirements and necessary corrective action should enterococci be detected (see A.4).
A.4 Monitoring considerations
Authorities and water utilities need to be aware that fecal indicator bacteria are used to provide a check of the performance of the barriers in place for the production of safe drinking water. They are not intended to serve as a rapid indicator of system operational issues, but they can alert water utilities to serious water quality problems that require action. Ideally, water utilities wishing to further achieve their objective of minimizing risk and assuring drinking water quality should focus their strategies on process management improvements. For example, the development of a water safety plan can help identify priority drinking water system hazards and risks as well as the monitoring tools needed for verifying that the system is delivering drinking water of the desired level of quality.
For any drinking water system, sampling frequency will vary based on the goals of the monitoring program as discussed in B.3.3. The presence of enterococci provides evidence of the potential vulnerability of the source water or treated and distributed water to fecal contamination. Detection should lead to further action including an evaluation of potential sources or causes and of the capability of the barriers in place to achieve safe-drinking water. When enterococci are detected in a drinking water sample, confirmation, notification and corrective actions similar to those outlined in the guideline technical document for E. coli should be put in place.
Part B. Supporting information
B.1 Description, sources, and survival
B.1.1 Description and sources
Enterococci are members of the bacterial genus, Enterococcus. They are Gram-positive, round-shaped bacteria that occur singly, in pairs or short chains.
The classification of enterococci has evolved over the years. Enterococci were first considered a specific division of the genus Streptococcus that met criteria of being able to: grow between 10 and 45°C, survive heating for 30 minutes at 60°C, and grow at pH 9.6 and at 6.5% sodium chloride. Enterococci have also been referred to as fecal streptococci since they were initially found in intestines of animals. The development of molecular methods led to the designation of Enterococcus as a new genus in 1984 (Fisher and Phillips, 2009).
The genus is currently thought to comprise 30-plus species classified into 5–6 major groups (E. faecalis, E. faecium, E. avium, E. gallinarum, E. italicus, and E. cecorum) (Svec and Devriese, 2009; Byappanahalli et al., 2012a). However, much uncertainty still exists regarding the true number of species, their groupings and the scope of their habitats (Del Mar Lleò et al., 2005). The terms, enterococci, fecal streptococci and intestinal enterococci are encountered in the drinking water literature, but for practical purposes these can be considered largely synonymous and interchangeable (Del Mar Lleò et al., 2005; Byappanahalli et al., 2012a).
Enterococci occur naturally in the intestines of humans and a range of animals, including mammals, birds, reptiles and insects. Human and animal feces are a habitat for virtually all enterococci species (Byappanahalli et al., 2012a). Although enterococci are members of the natural fecal flora, occasionally some have also been implicated in opportunistic infections. These have occurred at locations outside the intestines under conditions where immunity has been suppressed, where tissue injuries have occurred or where the body’s normal flora has been disrupted as a result of use of prescription antibiotics (Tendolkar et al., 2003). Consumption of treated drinking water has not been reported as a route of exposure leading to infection.
Enterococci have also been detected in diverse environmental habitats (Byappanahalli et al., 2012a; Staley et al., 2014). These have included plants, flowers, vegetables, cereals and grasses (Mundt et al., 1962; Müller et al., 2001; Ott et al., 2001; Sánchez Valenzuela et al., 2012); freshwater and marine water sand, soil and sediments (Obiri-Danso and Jones, 2000; Ran et al., 2013); and mats of the green algae Cladophora, decaying seaweed and submerged aquatic vegetation (Anderson et al., 1997; Whitman et al., 2003; Badgley et al., 2010; Byappanahalli et al., 2012a).
Concentrations in human and animal feces typically fall in the range from 103-107 cells per gram (Ashbolt et al., 2001; Leclerc et al., 2001; Ervin et al., 2013). Numbers in animal species can vary considerably and some studies have found higher enterococci numbers in the feces of farm animals and domestic pets than in human feces (Ervin et al., 2013; Masters et al., 2015). In general, numbers of enterococci in human and animal feces are lower than E. coli numbers by one to a few orders of magnitude (on a log10 scale) (Donnison, 1992; Cabral, 2010; Ervin et al., 2013, Boehm and Sassoubre, 2014). In general, concentrations of enterococci in temperate lakes and rivers are typically less than 103 colony forming units (CFU) per 100 mL (Jenkins et al., 2005; Ran et al., 2013). Concentrations can be elevated in response to rainfall events, with surface water samples containing 104–105 CFU/100 mL having been recorded (Haack et al., 2003; Wilkes et al., 2009; Nnane et al., 2011). Concentrations exceeding 102 CFU per 100 mL have been reported for contaminated groundwater sources (Atherholt et al., 2003; Schneeburger et al., 2015). Counts of 103-104 organisms/g have been recorded in sands, soils and beach substrates, such as algae (Whitman et al., 2003; Ran et al., 2013).
The species most commonly identified from enteric and environmental niches are E. faecalis and E. faecium (Staley et al., 2014). E. faecalis and E. faecium are the predominant species encountered in human and animal feces and sewage. Other species commonly isolated from human fecal material, but in lower numbers include E. durans, E. hirae, E. gallinarum, and E. avium (Poucher et al., 1991; Moore et al., 2008; Staley et al., 2014). Several species (e.g., E. cecorum, E. columbae, E. casseliflavus, E. durans, E. hirae, E. mundtii) have also been identified as common members of the normal intestinal flora of various animal species (Staley et al., 2014; Splichalova et al., 2015; Medeiros et al., 2017; Beukers et al., 2017).Members of the genus that have been found among plants have included E. faecalis E. faecium E. camelliae, E. casseliflavus, E. mundtii, and E. sulfureous (Collins et al., 1986; Müller et al., 2001; Ott et al., 2001; Byappanahalli et al., 2012a, Staley et al., 2014). Numerous enterococci species have been recovered from sands and soils (Byappanahalli et al., 2012a; Ran et al., 2013).
Surface water and groundwater investigations have demonstrated that other enterococci species may be encountered at frequencies similar to or exceeding those of E. faecium or E. faecalis (Moore et al., 2008; Suzuki et al., 2012; Furtula et al., 2013). Moore et al. (2008) observed that E. casseliflavus was the dominant species in urban runoff, while E. faecium, E. faecalis and E. hirae were dominant in sewage samples. Only a few studies have characterized the enterococci species detected in municipal scale drinking water supplies and groundwater sources. Available reports have consistently identified that the feces species E. faecalis and E. faecium are the two species detected most often in these sources (Sinton and Donnison, 1994; Celico et al., 2004; Grammenou et al., 2006; Jackson et al., 2012; Peter et al., 2012). Other enterococci species have been detected, but typically less often (Sinton and Donnison, 1994; Jackson et al., 2012).
Enterococci have been found in the environment in the absence of known sources of fecal contamination (Whitman et al., 2003; Byappanahalli et al., 2012a, 2012b) However, enterococci can still be regarded as indicators of fecal pollution for most practical purposes (WHO and OECD, 2003; AWWA, 2017). The detection of enterococci in a groundwater source or drinking water sample provides a strong indication of recent fecal pollution and, at minimum, provides evidence of a possible pathway for the entry of fecal contamination. Evaluation of the species present and possible origins can be undertaken to further understand system issues.
B.1.2 Survival
Numerous factors affect the survival of indicator bacteria in water environments including temperature, exposure to sunlight, presence and types of other microflora, availability of nutrients, oxygen levels, interactions with soils and related media, and the type of water involved (e.g., groundwater, surface water, treated distribution water). Enterococci can persist in water from as little as a few hours to as long as a few weeks. In habitats that can provide nutrients and protection from environmental stresses (e.g., soils, sand, and masses of aquatic vegetative material), survival of enterococci can be prolonged to the scale of months (Davies et al., 1995; Whitman et al., 2003; Pote et al., 2009).
Certain strains of enterococci may be successful at establishing populations in environmental habitats where extended persistence and potentially growth may occur (Ishii and Sadowsky, 2008; Byappanahalli et al., 2012b, Ran et al., 2013; Staley et al., 2014). Similar findings have been observed with other bacteriological indicators of fecal contamination, such as E. coli (Whitman et al., 2003; Byappanahalli et al., 2012b; Jang et al., 2017). How these environmental habitats contribute to indicator occurrence in drinking water sources is poorly understood, and further study in this area is needed (Staley et al., 2014). Available data suggests that where they have been detected, enterococci populations represent only a small part of the total soil microflora (Byappanahalli et al., 2006; 2012a). Nevertheless, further study is needed to better understand the ecology of fecal indicators in soil and related environments (Byappanahalli et al., 2012a, 2012b). Compared to soils or masses of vegetative material, most water environments provide conditions that are less protective and less nutrient-rich. Growth of enterococci in water habitats has not been demonstrated (Boehm and Sassoubre, 2014; Staley et al., 2014).
Historically, enterococci have been regarded as being more resistant to environmental stresses, such as increased salinity or desiccation, than the traditional fecal indicator bacteria, E. coli or thermotolerant coliforms (WHO, 1997, 2004). It has been proposed that this may be due in part to their thicker Gram-positive cell wall (Byappanahalli et al., 2012a). A number of studies have reported enterococci being capable of surviving longer than E. coli in marine waters (Lessard and Sieburth, 1983; Sinton et al., 1994; Bordalo et al., 2002; Fujioka and Yoneyama, 2002; Sinton et al., 2002). Enterococci have also shown a greater tolerance to desiccation than E. coli in studies of indicator survival in beach sand and tropical soils (Byappanahalli and Fujioka, 2004; Mika et al., 2009). In fresh waters, differential survival rates have been observed, with some studies showing longer survival for E. coli/fecal coliforms and others showing longer survival for enterococci (Sinton et al., 2002; Anderson et al., 2005; Deller et al., 2006; Fisher et al., 2012). As a result, strong conclusions about the comparative survival of enterococci and E. coli in fresh waters cannot be made. Studies evaluating indicator survival in groundwater have in general reported slower inactivation rates for enterococci compared to E. coli or fecal coliforms (McFeters et al., 1974; Keswick et al., 1982; Bitton et al., 1983; John and Rose, 2005). This data suggests that enterococci may persist longer than these indicators in groundwater.
Under the stresses of the water environment, enterococci can enter a viable but non-culturable (VBNC) state where they do not grow on laboratory media, but are otherwise alive and capable of resuscitation when conditions become favourable (Boehm and Sassoubre, 2014; Ramsey et al., 2014). The VBNC state is a primary survival strategy for bacteria that has been observed with numerous species, including those relevant to drinking water (Ramsey et al., 2014). The implications of the VBNC state on the use of standard microbiological methods for testing drinking water quality are not fully understood (van der Kooij and van der Wielen, 2014). Overall, enterococci can be considered to have a life span on the same order as most waterborne bacterial pathogens. It is generally agreed, however, that enterococci do not survive as long in the environment as waterborne pathogenic protozoa or viruses (Sinton et al., 2002; Medema et al., 2003).
B.2 Significance in water
B.2.1 Occurrence in surface water
Investigations of indicator concentrations in river catchments subject to agricultural and municipal waste inputs have shown strong correlations between enterococci and E. coli detections, with similar average and maximum values reported for these organisms (Brookes et al., 2005; Wilkes et al., 2009; Niño de Guzmán et al., 2012; Nnane et al., 2011, Yard et al., 2014).
B.2.2 Occurrence in groundwater
Drinking water regulations in the province of Quebec require monitoring for enterococci in the following situations: non-disinfected groundwater systems considered vulnerable (i.e., where fecal contamination source is present); for disinfected groundwater systems that do not meet the minimum 4-log removal/inactivation requirements for enteric viruses; and for non-disinfected groundwater systems where fecal coliforms or E. coli have been detected in the distribution (MDDELCC, 2016). Data provided by the Quebec Ministry of the Environment and the Fight Against Climate Change for the period from 2010-2014 showed that more than 33% of the 150-plus systems testing for enterococci reported at least one positive detection (MDDELCC, 2016). In comparison, positive detections for E. coli were reported at 18% of 200-plus stations (MDDELCC, 2016). Overall, only a small percentage of tests for enterococci and E. coli at all of these systems were positive (2.4% and 1.4% respectively). In a study conducted in Quebec on the impacts of manure application practices in the watershed on groundwater quality, the frequency of detection of enterococci and E. coli among all groundwater samples (n=1,260) was 5.8% and 1.5% respectively (Government of Quebec, 2004). For both indicators, the frequency of detection in groundwater samples from watersheds where manure was applied was not significantly different from that reported for samples from the non-impacted control areas.
Reviews of drinking water reports prepared by European Union (EU) Member States and drinking water authorities for England and Ireland revealed that small systems were more frequently non-compliant for enterococci test results than were larger water utilities (KWR, 2012; European Commission, 2015; DWI, 2016; EPA, 2016a, 2016b).
Some groundwater investigations have shown evidence of greater persistence of enterococci compared to thermotolerant coliforms or E. coli in contaminated aquifers (Sinton and Donnison, 1994; Roser et al., 2005; Naclerio et al., 2008; Schneeberger et al., 2015). In studies of septic system impacts on nearby groundwater sources, enterococci populations showed more gradual declines and were present in higher numbers with increasing distance from the contamination source when compared to E. coli or thermotolerant coliform populations (Sinton and Donnison, 1994; Schneeberger et al., 2015).
B.2.3 Occurrence in treated water and in the distribution system
Data on the detection of enterococci in the treated water and distribution systems of municipal-scale drinking water supplies in North America is limited. In the EU, Member States' drinking water reports for large systems have shown that although the majority of states report greater than 99% compliance with respect to microbiological parameters under the Drinking Water Directive, a large number of water supply zones report at least one incidence of non-compliance related to enterococci and E. coli sampling results (KWR, 2012; European Commission, 2015). Many annual reports provided by member states show enterococci detections occurring more frequently and in a greater number of water supply zones than E. coli detections (KWR, 2012; European Commission, 2015).
Studies involving municipal drinking water distribution systems have detected enterococci in the absence of E. coli, or reported more frequent detection in samples collected from dead ends or where residual chlorine concentrations were greater than 0.1 mg/L (Mendez et al., 2004; Batté et al., 2006).
During an examination of rural drinking water systems in Alabama, Wedgworth et al. (2015) observed that the number of samples that were positive for enterococci was significantly greater than that for E. coli at all locations sampled (well, post-treatment, post-storage, in-line and end line).
Detection of enterococci in biofilms in full-scale municipal systems was infrequent and in low numbers when present (Lee and Kim, 2003; Batté et al., 2006; Långmark et al., 2007). Although in some instances enterococci can be detected in biofilms in distribution systems, they do not seem to be a significant component of the biofilm matrix.
There is some data available regarding detection of enterococci (and other fecal indicators) at the point of drinking water consumption. In the United Kingdom (U.K.), samples to assess regulatory compliance are taken from consumer’s taps. Data from drinking water authorities for the U.K. and Ireland for the years 2010-2014 showed that for large public supplies, the vast majority of samples per year were compliant with the regulatory standard of 0 enterococci per 100 mL (DWI, 2016; DWQR, 2016a, 2016b; EPA, 2016a, 2016b; Northern Ireland Water, 2016). Across the various jurisdictions over this time, the number of non-compliant samples per country per year ranged from a minimum of 0 to a maximum of 11. Although the number of samples collected for enterococci was typically less than that collected for E. coli, enterococci was at times detected at a greater relative frequency (DWI, 2016; DWQR, 2016a, 2016b; EPA, 2016a, 2016b; Northern Ireland Water, 2016).
B.2.4 Role of enterococci as an indicator
The adoption of a risk-based approach, such as a source-to-tap or a water safety plan approach, is essential to the effective management of drinking water systems (CCME, 2004; WHO, 2017; Health Canada, 2013; Government of Alberta, 2015). Current Canadian drinking water guidelines encourage the adoption of a source-to-tap approach to produce clean, safe, and reliable drinking water. This approach includes the protection of source water, the use of appropriate and consistently effective treatment, a well-maintained distribution system, qualified personnel, routine verification of drinking water quality, and communication and public education.
E. coli and total coliforms are bacterial indicators that are used to verify water safety and changes in water quality, respectively. Enterococci are an additional bacteriological indicator of fecal contamination that can also be used under a source-to-tap approach to demonstrate that the drinking water system is producing water that is microbiologically acceptable. Support for the use of multi-indicator approaches for verifying drinking water quality is provided in the literature (WHO and OECD, 2003; WHO, 2005, AWWA, 2017).
The current understanding is that enterococci are a less fecal-specific indicator of contamination compared to E. coli (WHO and OECD, 2003; Byappanahalli et al., 2012a; Jang et al., 2017). However, the enterococci group of organisms possess characteristics that provide advantages when used as a fecal contamination indicator. Studies have shown that enterococci are somewhat more resistant to disinfectants commonly used in the drinking water industry when compared to E. coli (see Section B.4). Enterococci have also been shown to survive better and therefore be transported further than E. coli and thermotolerant coliforms in certain environments (e.g., marine waters, groundwater) (Keswick et al., 1982; Bitton et al., 1983 Sinton and Donnison, 1994; Naclerio et al., 2008; Schneeberger et al., 2015). Thus, because of their longer persistence, enterococci may be detected in cases where E. coli has not been detected.
Any decisions regarding including enterococci as a monitoring parameter in a regulatory or legislative context should be made by the responsible drinking water authority in cooperation with drinking water utilities. For water utilities, the decision to monitor for enterococci should be informed by a system-specific assessment.
It is important for water utilities to remember that the purpose of routine monitoring for bacteriological indicators is to provide a check that the drinking water system is under control and performing adequately. Indicator detection can alert water utilities to serious water quality problems that require further evaluation and action. However, they are not intended to serve as a rapid indicator of system operational issues. Information from all routine operational monitoring parameters (e.g., bacteriological indicators, disinfection measurements, turbidity) should be used together to inform assessments of drinking water safety and to support decision making.
B.2.4.1 Source water monitoring
For surface water sources, data suggests that enterococci would not provide a more sensitive indication of fecal contamination or pathogen presence than E. coli (Brookes et al., 2005; Wilkes et al., 2009, Yard et al., 2014).
For groundwater sources, evidence suggests that wells identified as being susceptible to fecal contamination (e.g., via source water assessments or historical data) are a setting where adding enterococci into a monitoring plan may provide benefits. Data from monitoring programs for groundwater showed that enterococci testing indicated additional instances of fecal contamination that were not captured by E. coli testing (Payment and Locas, 2005; MDDELCC, 2016). Fout et al. (2017) also found that enterococci may be a more successful predictor of wells vulnerable to pathogen contamination than E. coli in hydrogeologically susceptible settings.
Detection of enterococci in a groundwater well indicates the presence of a possible pathway for fecal contamination. Further actions should include an evaluation of the barriers in place to achieve safe-drinking water and the consideration of corrective actions for non-disinfected systems and systems unable to meet disinfection requirements in place.
B.2.4.2 Treated and distributed water monitoring
Enterococci are somewhat more resistant to drinking water disinfectants that E. coli, but both are readily inactivated at doses recommended for drinking water disinfection. (See Section B.4). The available data is not sufficient to show that enterococci testing of water immediately after treatment or leaving the treatment plant would provide significantly improved information over other indicator testing on treatment effectiveness.
With respect to distribution system monitoring, the evidence to date suggests that enterococci may be a useful indicator of post-treatment contamination (Mendez et al., 2004; Batté et al., 2006; KWR, 2012, European Commission, 2015; DWI, 2016; DWQR, 2016b). Datasets from distribution system monitoring programs and full-scale studies have demonstrated that the frequency of detection of enterococci can be greater than for E. coli (Mendez et al., 2004; Batté et al., 2006; KWR, 2012; DWI, 2016; DWQR, 2016b EPA, 2016a, 2016b). Monitoring programs for determining water quality following the disinfection of newly installed or repaired water mains are one particular area where adding enterococci testing may be valuable (WHO and OECD, 2003). Some standards governing the installation of drinking water require enterococci testing following the disinfection of new pipelines (BNQ, 2018).
Well-designed, operated and properly maintained treatment and distribution systems should consistently produce drinking water with no detectable enterococci. Therefore, the presence of enterococci in treated, distributed water indicates inadequate treatment or post-treatment contamination. Further actions should include a response similar to that recommended for E. coli. This can include notification, corrective actions and the use of boil water advisories.
B.2.5 Relationships with pathogenic microorganisms
Numerous studies have investigated linkages between the presence of indicator bacteria and the detection of specific fecal bacterial, viral or protozoan pathogens in surface water sources. Individual studies have occasionally observed a correlation between the presence of either enterococci or E. coli (or other indicators) and the detection of a specific pathogen, however the relationships are generally weak (Brookes et al., 2005, Wilkes et al., 2009). Studies in groundwater sources have been fewer in number and have shown weak correlations at best (Borchardt et al., 2003; Locas et al., 2007, 2008; Pitkänen et al., 2011; Hynds et al., 2014). Researchers studying microbiological quality in Canadian drinking water municipal wells observed that total coliforms and, to a lesser extent, E. coli were better indicators than enterococci in predicting the presence of human enteric viruses (Locas et al., 2007, 2008). Reviews of the body of evidence on this subject have concluded that the probability of a correlation with the presence of fecal pathogens in water samples was not higher with enterococci than with E. coli (Payment and Locas, 2011; Wu et al., 2011; Fout et al., 2017). Although the presence of enterococci is not necessarily associated with the presence of specific pathogens, in some studies of gastrointestinal illness and indicator presence in small drinking water supplies, data on the presence of enterococci fit the statistical models better than the E. coli data (Borchardt et al., 2003; Risebro et al., 2012). As well, studies involving recreational waters have reported linkages between gastrointestinal and acute febrile respiratory illnesses and enterococci concentrations (Kay et al., 1994; Fleisher et al., 1996; Wade et al., 2006, 2010; Heaney et al., 2012, 2014).
Direct correlations between indicator concentrations and the type or concentration of any pathogen should not be expected. Within a watershed, indicators and pathogens may come from multiple different sources, and once discharged into water sources experience different dilution, transport, and inactivation rates (Wilkes et al., 2009; Payment and Locas, 2011). Despite the lack of direct correlations with specific pathogens, the presence of enterococci in surface or groundwater sources generally indicates fecal contamination and thus a potential health risk, regardless of whether or not specific pathogens are observed (Wu et al., 2011).
B.3 Analytical methods
Enterococci are detectable by methods that require basic bacteriological laboratory facilities. The responsible drinking water authority should be consulted about requirements regarding the use of accredited laboratories and any other requirements that may apply. When purchasing laboratory services or selecting analytical methods for analysis to be performed in-house, water utilities should consult with the analytical laboratory or manufacturer for information on method sensitivity and turnaround time.
Most culture media methods for enumerating enterococci are based on the detection of the activity of the enzyme β- glucosidase (esculinase) which is present in the large majority of enterococci species and strains. Methods also take advantage of biochemical characteristics specific to the Enterococcus genus and use media additives and incubation temperatures to inhibit the growth of background microorganisms and differentiate enterococci from other Gram-positive bacteria.
Media have been designed to identify enterococci in water samples without identifying the species (Leclerc et al., 1996; APHA et al., 2017). It is important to use validated or standardized methods to make correct and timely public health decisions (Leclerc et al., 1996). Additional verification or confirmation steps, if required, will prolong the time required to obtain results (APHA et al., 2017).
B.3.1 Culture-based methods
Standard Methods for the Examination of Water and Wastewater lists methods for enumeration of enterococci via the membrane filtration (MF) technique, the multiple tube fermentation (MTF) technique and a fluorogenic substrate test (APHA et al., 2017). Membrane filtration methods are considered to represent the current “gold standard” for enterococci water-quality assessments (Byappanahalli et al., 2012a). The fluorogenic substrate methods have the advantage of ease of use and lesser equipment requirements. The MTF approach is recommended as an option when waters are highly turbid (APHA et al., 2017). Formulations of the fluorogenic substrate are available commercially for use in a MTF, multi-well or presence-absence format (APHA et al., 2017). International Organization for Standardization (ISO) Method 7899-2 is the specified method of analysis for enterococci under the EU Council Directive, though member states can use alternative equivalent methods (EU, 1998; ISO, 2018). A list of published standardized methods is provided in Table 1.
Organization and method | Media | Basis for detection | Turnaround time | Test procedures |
---|---|---|---|---|
Multiple tube fermentation (MTF) | ||||
SM 9230 Ba U.S. EPA -N/Ab |
Azide dextrose broth- bile esculin azide agar (2-step method) |
β-glucosidase enzyme | 48-72 h | Azide dextrose broth (35°C, 24-48 h); Bile esculin azide agar (35°C, 24 h) |
Membrane filtration (MF) | ||||
SM 9230 Ca U.S. EPA - N/Ab |
mE-EIA (2-step method) | β-glucosidase enzyme | 48 h | mE agar (41°C, 48 h); EIA media (41°C, 20 min.) |
SM 9230 Ca U.S. EPA – 1600c |
mEI (1-step method) | β-glucosidase enzyme | 24 h | mEI agar (41°C, 24h) |
SM 9230 Ca U.S. EPA - N/Ab |
mEnterococcus (1-step method) | Growth on target media | 48 h | mEnterococcus agar (35°C, 48 h) |
ISO 7899-2d | Slanetz and Bartley agar-bile esculin azide agar (2-step method) | β-glucosidase enzyme | 46 h | Slanetz and Bartley agar (36°C, 44 h); Bile esculin azide agar (44°C, 2h) |
Fluorogenic substrate test (MTF, multi-well, presence-absence) | ||||
SM 9230 Da U.S. EPA - N/Ab |
Enterolert® medium (1 step method) | β-glucosidase enzyme | 24 h | Enterolert® medium (41°C, 24h) |
a APHA et al. (2017), b U.S. EPA (2017), c U.S. EPA (2006b), d ISO (2018). |
Variability in the performance of test methods has been observed during laboratory testing. Correlation coefficients ranging from 0.68 to 0.93 have been reported in studies comparing the performance of the commercial fluorogenic substrate test and different MF methods in various water matrices (fresh and marine bathing waters, surface water, and treated drinking water) (Fricker and Fricker, 1996; Abbot et al., 1998; Eckner, 1998; Kinzelman et al., 2003). In some studies, the commercial test was shown to be of similar or greater sensitivity (Fricker and Fricker, 1996; Eckner, 1998; Kinzelman et al., 2003); whereas in others the MF methods were shown to be more sensitive (Adcock and Saint, 2001; Heiber et al., 1998; Maheux et al., 2009).
A study compared the ability of three β-glucosidase-based commercially available test methods to detect 110 different Enterococcus strains from diverse origins (Maheux et al., 2009). Detection performances reported for a commercial fluorogenic substrate method and two membrane filtration methods were 68.3%, 83.2% and 88.1% respectively. With strains of the primary fecal species E. faecalis and E. faecium, detection performance for all three methods was close to 90% or greater (Maheux et al., 2009). In a comparative analysis of these same three methods for the detection of enterococci in well water samples collected in the Quebec City region, rates of detection reported were 3.0%, 5.5% and 11.5% respectively (Maheux et al., 2012). Standardized methods for the detection of enterococci have been validated against established reference methods to ensure that the procedure performs to an acceptable level (APHA et al., 2017). Nevertheless, there is a need to continually evaluate the efficacy of enterococci test methods, and to improve their sensitivity and specificity.
B.3.2 Molecular methods
Molecular methods for the detection of enterococci in natural waters have been developed by the (U.S. EPA (2015a, 2015b). However, these are currently not included on the list of methods approved under the Clean Water Act for the microbiological testing of ambient waters (U.S. EPA, 2019). The U.S. EPA Methods 1609.1 and 1611.1. both describe procedures for the detection of DNA from enterococci bacteria based on the amplification and detection of a specific region of the large subunit ribosomal ribonucleic acid (RNA) gene (23S rRNA) from these organisms.
No standardized methods have been developed for the detection of enterococciin drinking water using molecular techniques (APHA et al., 2017, ISO, 2019, U.S. EPA, 2019). The most significant challenge associated with drinking water analysis is the stricter limit for indicator organism presence, and therefore the need for method sensitivity at very low concentrations. More work is needed in this area to develop standardized methods that can be used accurately, reliably and affordably. Maheux et al. (2011) have described a molecular method combining microbial particle concentration and recovery, whole genome amplification for Enterococcus spp. (23S rRNA gene targets) and a multiplex real-time PCR assay with primers and probes specific to targets for E. faecalis (mtlf gene) and E. faecium (ddl gene). The authors reported detection of as few as 4.5 enterococci cells per 100 mL in less than 5 h with this method and further stated it should theoretically be able to detect 1 CFU of enterococci per 100 mL. Pitkanen et al. (2017) have described a method, which uses an RT-qPCR (reverse-transcription quantitative PCR) assay to target rRNA transcripts from the enterococci 23S rRNA gene. Further validation is needed, but the study data suggested this approach significantly increased detection sensitivity over DNA-based qPCR assays targeting the 23S rRNA gene itself (Pitkanen et al., 2017).
Similar to culture methods, current PCR methods using ribosomal RNA gene targets are capable of detecting the Enterococcus genus, but are not able to distinguish individual species (Ryu et al., 2013).
Molecular methods are also being explored for the rapid identification of enterococci species detected in drinking water analyses and for understanding the diversity and origins of enterococci present in environmental waters (Ryu et al., 2013; Weigand et al., 2014; Park et al. 2017). Park et al. (2017) has described a multiplex-PCR method, which uses species-specific primers to identify several Enterococcus species. Continued research in these areas is necessary.
B.3.3 Sampling for enterococci
Proper procedures for collecting samples must be observed to ensure that the samples are representative of the water being examined. Detailed instructions on the collection of samples for bacteriological analysis are given in Standard Methods for the Examination of Water and Wastewater (APHA et al., 2017). The analytical laboratory should be consulted about requirements for sample collection and transport. Ideally, the interval between collection of the sample and the beginning of its examination should not exceed 24 hours (Bartram and Rees, 2000), and analysis within 8 hours is recognized as the preferred time interval (Bartram and Rees, 2000; APHA et al., 2017). In remote areas, up to 48 hours may be an acceptable time interval; however, the implications of the extended holding time should be discussed with the responsible authorities. When long holding times are anticipated, onsite testing with standardized commercialized test methods (see Table 1) in combination with appropriate training and quality control procedures is a viable analytical option. Water utilities should first consult with the responsible drinking water authority about the acceptability of this practice and any other requirements that may apply.
A minimum volume of 100 mL of water should be examined to obtain a reliable estimate of the number of organisms (using MTF, MF or presence-absence). Examination of larger volumes can increase both the sensitivity and the reliability of the test. Smaller volumes, dilutions, or other MTF combinations may be more appropriate for waters of poor quality.
When determining sampling frequency requirements for municipal scale systems, the application of a universal sampling formula is made impossible by basic differences in factors such as source water quality, adequacy and capacity of treatment, and size and complexity of the distribution system (WHO, 1971, 1976, 2004).
The number of samples for enterococci testing can also vary depending on the expected goals of the monitoring strategy. If the monitoring goal is routine fecal indicator testing to verify the quality of the source, the adequacy of drinking water treatment or the microbial condition of the distribution system, sampling at a frequency similar to that used for other microbiological indicators such as E. coli and total coliforms is recommended.
If the monitoring goal is for periodic inspections of drinking water source (e.g., groundwater source) or distribution system water quality in response to suspected or identified issues, periods of targeted sampling can be conducted until the issue is addressed.
Specific requirements for the sampling frequency and location of sampling points for enterococci testing should be established by the responsible authority in cooperation with drinking water utilities. Sampling points may include those used for E. coli and total coliforms testing for convenience. In groundwater monitoring programs, event-based monitoring (i.e., monitoring after an event that can pose an increased risk of fecal contamination for vulnerable systems) can also be valuable for detecting problems. These events can include spring snowmelt and runoff, heavy rainfalls, flooding and droughts. For distribution systems, requirements for microbiological testing in response to disinfection procedures for newly installed or repaired water mains are typically available from provincial/territorial jurisdictions. Further guidance on procedures and best practices can be found elsewhere (AWWA, 2005, Kirmeyer et al., 2014).
Simulation studies have shown that it is very difficult to detect a contamination event in a distribution system unless the contamination occurs at the treatment plant, in a trunk main, in a reservoir, or is of long duration at a high concentration (Speight et al., 2004; van Lieverloo et al., 2007). In addition, with microbiological indicator testing, the low rate of positive samples means that it can be difficult to see statistically significant differences in positive rates, for example, before and after a corrective action, unless a very large number of samples are evaluated (Rosen et al., 2009). There are limited demonstrations evaluating effective monitoring strategies, and additional statistical and field work are needed that simultaneously consider the parameters of sample volume, monitoring frequency, detection method, false/true positives and negatives, and cost. These limitations highlight the importance of implementing a source-to-tap approach or water-safety-plan approach for ensuring the microbiological quality of the drinking water.
B.4 Treatment considerations
The primary goal of treatment is to reduce the presence of disease-causing organisms and associated health risks to an acceptable or safe level.
There has been limited data published on the effectiveness of various drinking water treatment technologies (i.e. filtration or disinfection processes) specific to the removal and inactivation of enterococci. Overall, physical removal methods (including coagulation, flocculation, sedimentation, slow or rapid sand filtration and direct filtration with or without filtration aid) can accomplish 1–4 log removal of indicator organisms (E. coli, coliforms, enterococci) (Payment et al., 1985; Smeets et al., 2006). Membrane filtration technologies are also capable of removing 4 log to greater than 6 log of bacteria (Smeets et al., 2006). Disinfectants commonly used in the drinking water industry, such as chlorine, chloramine, chlorine dioxide, ozone, and ultraviolet (UV) light, are known to be effective against the enterococci group. Each of the above agents has demonstrated the ability to produce greater than a 4 log inactivation of enterococci in laboratory or pilot-scale experiments (Chang et al., 1985; Fujioka et al., 1986; Harris et al., 1987; Rice et al., 1993; Restaino et al., 1995; Wiedenmann et al., 1997; Grunert et al., 2018; Stange et al., 2019).
Scientific studies have provided some evidence of the enterococci group being more resistant to inactivation with chlorine, monochloramine, chlorine dioxide and UV light as compared to E. coli (Chang et al., 1985; Harris et al., 1987; Rice et al., 1993; Perez Recuerda et al., 1998; Tavakoli et al., 2005; Grunert et al., 2018; Léziart et al., 2019; Stange et al., 2019). However, the limited data makes it difficult to draw strong conclusions). Developments on this topic will continue to be monitored. Still, it is generally accepted that enterococci responses to disinfection are of the same order of magnitude as E. coli (Hijnen et al., 2011). Overall, the evidence shows that enterococci are much more sensitive to chlorination than the enteric protozoans Giardia and Cryptosporidium, and more sensitive to UV inactivation than certain enteric viruses (Smeets et al., 2006; Hijnen et al., 2011; Health Canada 2019a; 2019b). Therefore, water that is treated to meet the guidelines for enteric viruses and enteric protozoa should have an acceptable bacteriological quality, including achieving enterococci concentrations of none detectable per 100 mL of water leaving the treatment plant. Further information on inactivation of specific protozoan and viral pathogens can be found in the guideline technical documents on enteric protozoa and enteric viruses (Health Canada, 2019a; 2019b).
In distribution systems, a disinfectant residual is needed to protect against bacterial regrowth and serve as a sentinel for water quality changes. Regular monitoring of distribution system water quality (e.g., microbial indicators, disinfectant residual, turbidity, pH) and having operations and maintenance programs in place (water mains cleaning, cross-connection control, replacements and repairs) are important for ensuring drinking water is distributed to consumers with minimum loss of quality (Kirmeyer et al., 2001, 2014).
Surface water is not recommended as a residential-scale water supply unless it is properly filtered, disinfected and monitored for water quality. Well water supplies can also be contaminated and may require treatment. Various options are available for treating source waters to provide high-quality pathogen-free drinking water. These include systems that rely on chlorine, UV light or filtration. Health Canada does not recommend specific brands of residential-scale drinking water treatment devices, but it strongly recommends that consumers look for a mark or label indicating that the device has been certified by an accredited certification body as meeting the appropriate NSF International (NSF)/American National Standards Institute (ANSI) standard. These standards have been designed to safeguard drinking water by helping to ensure the safety of material and performance of products that come into contact with drinking water. Treatment devices should be inspected and serviced in accordance with the maintenance schedule and manufacturer’s recommendations.
B.5 International considerations
The European Union (EU) Drinking Water Directive has included enterococci (originally fecal streptococci) as a microbiological drinking water parameter since 1980 (1980; 1998). The current EU Drinking Water Directive outlines the legislative requirements for all its Member States (1998). Under the directive, enterococci are categorized as a parameter for audit monitoring in the distribution system. Audit parameters are tested less frequently than check (i.e., routine monitoring) parameters; they are intended to provide information as to whether or not their specific parametric values are being complied with. The EU standard for enterococci is a value of zero per 100mL (1998).
The Guidelines for Drinking Water Quality by the World Health Organization (WHO) (2017) contain fact sheets on numerous microbial indicators, including intestinal enterococci. The guidance from WHO specifies that the intestinal enterococci group can be used as an index of fecal pollution. The presence of the group provides evidence of recent fecal contamination, and detection of intestinal enterococci should lead to consideration of further action. No guideline values are specified.
The Australian Drinking Water Guidelines provide fact sheets on numerous microbial indicators, including intestinal enterococci, but no guideline values are specified. In practice, enterococci can be used to assess source water quality, the adequacy of treatment, post-treatment ingress into the distribution system and the delivery of safe drinking water at consumer taps (NHMRC and NRMMC, 2017).
The U.S. EPA Ground Water Rule (2006a) lists enterococci as one of three possible microbiological indicators of fecal contamination, alongside E. coli and coliphages, which can be specified by the states. Under the rule, untreated ground water systems having a total coliform-positive sample must collect at least one sample from each ground water source and test for one of the state-specified indicators (referred to as triggered source water monitoring). Should a triggered sample test positive for the fecal indicator, the system must notify state authorities and the public and take corrective actions.
B.6 Research gaps
There has been a wealth of important research conducted that has advanced our knowledge of the Enterococcus genus and its applicability as an indicator of fecal contamination. Despite this, there are numerous information gaps that still need to be filled.
Currently, limited published Canadian data exists concerning the frequency of enterococci detection relative to other bacteriological indicators (E. coli, total coliforms) in response to contamination issues in groundwater sources and drinking water distribution systems. There is also a need for additional studies comparing the relative survival rates for E. coli and enterococciunder various drinking water treatment technologies with a variety of water qualities. Another important area for future research involves improving the understanding of the identity and sources of Enterococcus species found in groundwater sources and treated drinking water.
Lastly, continued work towards developing standardized molecular tests for enterococci detection and identification in drinking water would also be of great value.
Part C. References and acronyms
C.1 References
Abbott, S., Caughley, B. and Scott, G. (1998). Evaluation of Enterolert® for the enumeration of enterococci n the marine environment. N. Z. J. Mar. Freshwat. Res. 32: 505–513.
Adcock, P.W. and Saint, C.P. (2001). Development of glucosidase agar for the confirmation of water-borne Enterococcus. Water Res., 35 (17): 4243-4246.
Anderson, S.A., Turner, S.J. and Lewis, G.D. (1997). Enterococci in the New Zealand environment: Implications for water quality monitoring. Water Sci. Technol., 35 (11-12): 325–331.
Anderson, K.L., Whitlock, J.E. and Harwood, V.J. (2005). Persistence and differential survival of fecal indicator bacteria in subtropical waters and sediments. Appl. Environ. Microbiol., 71 (6): 3041–3048.
APHA, AWWA and WEF (2017). Standard methods for the examination of water and wastewater. 23rd edition. American Public Health Association, American Water Works Association and Water Environment Federation, Washington, DC.
Ashbolt, N.J., Grabow, W.O.K. and Snozzi, M. (2001). Indicators of microbial water quality. In Water quality—Guidelines, standards and health: Assessment of risk and risk management for water-related infectious disease. Fewtrell, L. and Bartram, J. (Eds.). IWA Publishing, London, United Kingdom, on behalf of the World Health Organization. pp. 289–315.
Atherholt, T., Feerst, E., Hovendon, B., Kwak, J. and Rosen, J.D. (2003). Evaluation of indicators of fecal contamination in groundwater. J. Am. Water Works Assoc., 95 (10): 119–131.
AWWA. 2005. AWWA Standard C651-05 - Disinfecting Water Mains. American Water Works Association. Denver, CO.
AWWA (2017). Water quality in distribution systems. AWWA manual of water supply practices M68. American Water Works Association, Denver, CO.
Badgley, B.D., Nayak, B.S. and Harwood, V.J. (2010). The importance of sediment and submerged aquatic vegetation as potential habitats for persistent strains of enterococci in a subtropical watershed. Water Res., 44 (20): 5857–5866.
Bartram, J. and Rees, G. (2000). Monitoring bathing waters. E & FN Spon, New York, New York.
Batté, M., Féliers, C., Servais, P., Gauthier, V., Joret, J.-C. and Block, J.-C. (2006). Coliforms and other microbial indicators occurrence in water and biofilm in full-scale distribution systems. Water Sci. Technol., 54 (3): 41–48.
Beukers, A.G., Zaheer, R., Goji, N., Amoako, K.K., Chaves, A.V., Ward, M.P. and McAllister, T.A. (2017). Comparative genomics of Enterococcus spp. isolated from bovine feces. BMC Microbiol., 17 (1), art. no. 52.
Bitton, G., Farrah, S. R., Ruskin, R. H., Butner, J. and Chou, Y. J. (1983). Survival of pathogenic and indicator organisms in groundwater. Ground Water, 21: 405-410.
BNQ (2018). BNQ 1809-300/2018. Travaux de construction — Conduites d’eau potable et d’égout — Clauses techniques générales. Bureau de normalisation du Québec. Montreal, Quebec.
Boehm, A.B. and Sassoubre, L.M. (2014). Enterococci as indicators of environmental fecal contamination. In Enterococci: From commensals to leading causes of drug resistant infection. Gilmore, M.S. et al., eds. Massachusetts Eye and Ear Infirmary, Boston, Massachusetts.
Borchardt, M.A., Chyou, P., DeVries, E.O. and Belongia, E.A. (2003). Septic system density and infectious diarrhea in a defined population of children. Environ. Health Perspect. 111:742–748.
Bordalo, A.A., Onrassami, R. and Dechsakulwatana, C. (2002). Survival of faecal indicator bacteria in tropical estuarine waters (Bangpakong River, Thailand). J. Appl. Microbiol., 93 (5): 864–871.
Brookes, J.D., Hipsey, M.R., Burch, M.D., Linden, L.G., Ferguson, C.M. and Antenucci, J.P. (2005). Relative value of surrogate indicators for detecting pathogens in lakes and reservoirs. Environ. Sci. Technol., 39 (22): 8614–8621.
Byappanahalli, M. and Fujioka, R. (2004). Indigenous soil bacteria and low moisture may limit but allow faecal bacteria to multiply and become a minor population in tropical soils. Water Sci. Technol., 50 (1): 27–32.
Byappanahalli, M.N., Nevers, M.B., Korajkic, A., Staley, Z.R. and Harwood, V.J. (2012a). Enterococci in the environment. Microbiol. Mol. Biol. Rev., 76: 685–706.
Byappanahalli, M.N., Yan, T., Hamilton, M.J., Ishii, S., Fujioka, R.S., Whitman, R.L. and Sadowsky, M.J. (2012b). The population structure of Escherichia coli isolated from subtropical and temperate soils. Sci. Total Environ., 417-418: 273-279.
Cabral, J.P.S. (2010). Water microbiology. Bacterial pathogens and water. Int. J. Environ. Res. Public Health 7(10): 3657–3703.
CCME (2004). From source to tap: guidance on the multi-barrier approach to safe drinking water. Produced jointly by the Federal-Provincial-Territorial Committee on Drinking Water and the CCME Water Quality Task Group. Canadian Council of Ministers of the Environment, Winnipeg, Manitoba. Available at www.ccme.ca/files/Resources/water/source_tap/mba_guidance_doc_e.pdf
Celico, F., Varcamonti, M., Guida, M. and Naclerio, G. (2004). Influence of precipitation and soil on transport of fecal enterococci in fractured limestone aquifers. Appli. Environ. Microbiol., 70 (5): 2843–2847.
Chang, J.C.H., Ossoff, S.F., Lobe, D.C., Dorfman, M.H., Dumais, C.M., Qualls, R.G. and Johnson, J.D. (1985). UV inactivation of pathogenic and indicator microorganisms. Appl. Environ. Microbiol., 49 (6): 1361–1365.
Collins, M.D., Farrow, J.A.E. and Jones, D. (1986). Enterococcus mundtii sp. nov. Int. J. Syst. Bacteriol. 36: 8–12.
Davies, C.M., Long, J.A.H., Donald, M. and Ashbolt, N.J. (1995). Survival of fecal microorganisms in marine and freshwater sediments. Appl. Environ. Microbiol. 61 (5): 1888–1896.
Deller, S., Mascher, F., Platzer, S., Reinthaler, F.F. and Marth, E. (2006). Effect of solar radiation on survival of indicator bacteria in bathing waters. Cent. Eur. J. Public Health, 14 (3): 133–137.
Del Mar Lleò, M., Signoretto, C. and Canepari, P. (2005). Chapter 13: Gram-positive bacteria in the marine environment. In Oceans and Health: Pathogens in the Marine Environment. Belkin, S. and Colwell, R.R. (Eds.) Springer. New York, New York. pp. 307–330.
Donnison, A.M. (1992). Enumeration of enterococci in New-Zealand waters and effluents. Environ. Technol., 13(8): 771–778.
DWI (2016). Drinking water 2010-2014 (annual reports). Drinking Water Inspectorate, DWI Publications. London, England. Available from: www.dwi.gov.uk
DWQR (2016a). Drinking water quality in Scotland. Private water supplies. 2010-2014. Drinking Water Quality Regulator for Scotland. Edinburgh, Scotland. Available from: www.DWQR.org.uk
DWQR (2016b). Drinking water quality in Scotland. Public water supplies. 2010-2014. Drinking Water Quality Regulator for Scotland. Edinburgh, Scotland. Available from: www.DWQR.org.uk
Eckner, K.F. (1998). Comparison of membrane filtration and multiple-tube fermentation by the Colilert and Enterolert methods for detection of waterborne coliform bacteria, Escherichia coli, and Enterococci used in drinking and bathing water quality monitoring in southern Sweden. Appl. Environ. Microbiol., 64 (8): 3079–3083.
EPA (2016a). Drinking water reports (2013-2014). Environmental Protection Agency. County Wexford, Ireland. Available from: www.epa.ie
EPA (2016b). The provision and quality of drinking water in Ireland – A report for the year (2010-2012). Environmental Protection Agency. County Wexford, Ireland. Available from: www.epa.ie
Ervin, J.S., Russell, T.L., Layton, B.A., Yamahara, K.M., Wang, D., Sassoubre, L.M., Cao, Y., Kelty, C.A., Sivaganesan, M., Boehm, A.B., Holden, P.A., Weisberg, S.B. and Shanks, O.C. (2013). Characterization of fecal concentrations in human and other animal sources by physical, culture-based, and quantitative real-time PCR methods. Water Res., 47 (18): 6873–6882.
EU (1980). Council Directive 80/778/EEC relating to the quality of water intended for human consumption. Available at https://eur-lex.europa.eu/legal-content/EN/TXT/?uri=CELEX%3A31980L0778
EU (1998). Council Directive 98/83/EC on the quality of water intended for human consumption. Available at https://eur-lex.europa.eu/legal-content/EN/TXT/?uri=CELEX%3A31998L0083
European Commission (2015). Synthesis report on the quality of drinking water in the EU examining the Member States' reports for the period 2008-2010 under Directive 98/83/EC. Brussels, Belgium. Available from: http://ec.europa.eu/environment/water/water-drink/reporting_en.html
Fisher, K. and Phillips, C. (2009). The ecology, epidemiology and virulence of Enterococcus. Microbiology, 155 (6):1749–1757.
Fisher, M.B., Iriarte, M. and Nelson, K.L. (2012). Solar water disinfection (SODIS) of Escherichia coli, Enterococcus spp., and MS2 coliphage: Effects of additives and alternative container materials. Wat. Res., 46 (6): 1745-1754.
Fleisher, J. M., Kay, D., Salmon, R. L., Jones, F., Wyer, M. D. and Godfree, A. F. (1996). Marine waters contaminated with domestic sewage: Nonenteric illnesses associated with bather exposure in the United Kingdom. Am. J. Public Health 86 (9): 1228–1234.
Fout, G.S., Borchardt, M.A., Kieke, B.A., Jr. and Karim, M.R. (2017). Human virus and microbial indicator occurrence in public-supply groundwater systems: Meta-analysis of 12 international studies. Hydrogeol. J., 25(4): 903-919.
Fricker, E.J. and Fricker, C.R. (1996). Use of defined substrate technology and a novel procedure for estimating the numbers of enterococci in water. J. Microbiol. Methods, 27: 207–210.
Fujioka, R.S. and Yoneyama, B.S. (2002). Sunlight inactivation of human enteric viruses and fecal bacteria. Water Sci. Technol., 46 (11-12): 291–295.
Fujioka, R.S., Dow, M.A. and Yoneyama, B.S.(1986). Comparative disinfection of indicator bacteria and poliovirus by chlorine dioxide. Water Sci. Technol., 18 (10):125-132.
Furtula, V., Jackson, C.R., Farrell, E.G., Barrett, J.B., Hiott, L.M. and Chambers, P. (2013). Antimicrobial resistance in Enterococcus spp. isolated from environmental samples in an area of intensive poultry production. Int. J. Environ. Res. Public Health, 10 (3): 1020–1036.
Government of Alberta (2015). A guidance framework for the production of drinking water safety plans. Alberta Environment and Parks. Edmonton, Alberta. Available from: http://environment.alberta.ca/apps/regulateddwq/DWSP.aspx
Government of Quebec (2004). Étude sur la qualité de l’eau potable dans sept bassins versants en surplus de fumier et impacts potentiels sur la santé. Bibliothèque nationale du Québec, 2004. ISBN 2-550-43506. Envirodoq ENV/2004/0310. Available from: www.santecom.qc.ca.
Grammenou, P., Spiliopoulou, I., Sazakli, E. and Papapetropoulou, M. (2006). PFGE analysis of enterococci isolates from recreational and drinking water in Greece. J. Water Health 4: 263–272.
Grunert, A., Frohnert, A., Selinka, H.-C., Szewzyk, R. (2018). A new approach to testing the efficacy of drinking water disinfectants. Int. J. Hyg. Envir. Heal., 221 (8):1124-1132.
Haack, S.K., Fogarty, L.R. and Wright, C. (2003). Escherichia coli and enterococci at beaches in the Grand Traverse Bay, Lake Michigan: Sources, characteristics, and environmental pathways. Environ. Sci. Technol., 37 (15): 3275–3282.
Harris, G.D., Adams, V.D., Sorensen, D.L. and Curtis, M.S. (1987). Ultraviolet inactivation of selected bacteria and viruses with photoreactivation of the bacteria. Water Res., 21 (6): 687–692.
Health Canada (2013). Guidance on the use of the microbiological drinking water quality guidelines. Water and Air Quality Bureau, Healthy Environments and Consumer Safety Branch, Health Canada, Ottawa, Ontario. (Catalogue No H144-12/2013E-PDF).
Health Canada (2019a). Guidelines for Canadian drinking water quality: Guideline technical document — Enteric protozoa: Giardia and Cryptosporidium. Water and Air Quality Bureau, Healthy Environments and Consumer Safety Branch, Health Canada, Ottawa, Ontario. Available at www.canada.ca/en/health-canada/services/environmental-workplace-health/reports-publications/water-quality.htm
Health Canada (2019b). Guidelines for Canadian drinking water quality: Guideline technical document — Enteric viruses. Water and Air Quality Bureau, Healthy Environments and Consumer Safety Branch, Health Canada, Ottawa, Ontario. Available at www.canada.ca/en/health-canada/services/environmental-workplace-health/reports-publications/water-quality.htm.
Heaney, C.D., Sams, E., Dufour, A.P., Brenner, K.P., Haugland, R.A., Chern, E., Wing, S., Marshall, S., Love, D.C., Serre, M., Noble, R. and Wade, T.J. (2012). Fecal indicators in sand, sand contact, and risk of enteric illness among beachgoers. Epidemiology, 23 (1): 95–106.
Heaney, C.D., Exum, N.G., Dufour, A.P., Brenner, K.P., Haugland, R.A., Chern, E., Schwab, K.J., Love, D.C., Serre, M.L., Noble, R. and Wade, T.J. (2014). Water quality, weather and environmental factors associated with fecal indicator organism density in beach sand at two recreational marine beaches. Sci. Tot. Environ, 497: 440–447.
Heiber, I., Frahm, E. and Obst, U. (1998). Comparison of four methods for the detection of fecal streptococci in water [Vergleich von vier Nachweismethoden für Fäkalstreptokokken in Wasser.]. Zentralblatt für Hygiene und Umweltmedizin = Int. J. Hyg. Environ. Med., 201 (4–5): 357–369.
Hijnen W.A.M. (2011). Quantitative methods to assess capacity of water treatment to eliminate micro-organisms. KWR Watercycle Research Institute. IWA Publishing, London, United Kingdom.
Hynds, P.D., Thomas, M.K. and Pintar, K.D.M. (2014). Contamination of groundwater systems in the US and Canada by enteric pathogens, 1990-2013: A review and pooled-analysis. PLoS ONE, 9 (5), art. no. e93301.
Ishii, S. and Sadowsky, M.J. (2008). Escherichia coli in the environment: Implications for water quality and human health. Microbes Environ., 23(2): 101-108.
ISO (2018) ISO ICS 07.100.20 – Microbiology of water. International Organization for Standardization. Geneva, Switzerland. Available at www.iso.org/ics/07.100.20/x/
Jackson, C.R., Furtula, V., Farrell, E.G., Barrett, J.B., Hiott, L.M. and Chambers, P. (2012). A comparison of BOX-PCR and pulsed-field gel electrophoresis to determine genetic relatedness of enterococci from different environments. Microb. Ecol., 64 (2): 378–387.
Jang, J., Hur, H., Sadowsky, M.J., Byappanahalli, M.N., Yan, T. and Ishii, S. (2017). Environmental Escherichia coli: Ecology and public health implications—a review. J. Appl. Microbiol., 123(3): 570-581.
Jenkins, T.M., Scott, T.M., Morgan, M.R. and Rose, J.B. (2005). Occurrence of alternative fecal indicators and enteric viruses in Michigan rivers. J. Great Lakes Res., 31 (1): 22–31.
John, D.E. and Rose, J.B. (2005). Review of factors affecting microbial survival in groundwater. Environ. Sci. Technol., 39 (19): 7345–7356.
Kay, D., Jones, F., Wyer, M.D., Fleisher, J.M., Salmon, R.L., Godfree, A.F., Zelenauch-Jacquotte, A. and Shore, R. (1994). Predicting likelihood of gastroenteritis from sea bathing: results from randomised exposure. The Lancet, 344 (8927): 905–909.
Keswick, B. H., Gerba, C. P., Secor, S. L. and Cech, I. (1982). Survival of enteric viruses and indicator bacteria in groundwater. J. Environ.Sci. Health, Part A: Environ. Sci. Eng. Toxic Hazard. Subst.Control. 17, 903-912.
Kinzelman, J., Ng, C., Jackson, E., Gradus, S. and Bagley, R. (2003). Enterococci as indicators of Lake Michigan recreational water quality: Comparison of two methodologies and their impacts on public health regulatory events. Appl. Environ. Microbiol., 69(1): 92–96.
Kirmeyer, G.J., Friedman, M., Martel, K., Howie, D., LeChevallier, M., Abbaszadegan, M., Karim, M., Funk, J. and Harbour, J. (2001). Pathogen intrusion into the distribution system. AWWA Research Foundation and American Water Works Association, Denver, Colorado.
Kirmeyer, G.J., Thomure, T.M., Rahman, R., Marie, J.L., LeChevallier, M.W., Yang, J., Hughes, D.M. and Schneider, O. (2014). Effective microbial control strategies for main breaks and depressurization. Water Research Foundation, Denver, Colorado.
KWR (2012). The quality of drinking water in the European Union 2005-2007. Synthesis report on the quality of drinking water in the Member States of the European Union in the period 2005-2007 Directive 98/83/EC Watercycle Research Institute. December. Available from: https://circabc.europa.eu/faces/jsp/extension/wai/navigation/container.jsp
Långmark, J., Storey, M.V., Ashbolt, N.J. and Stenström, T.-A. (2007). The effects of UV disinfection on distribution pipe biofilm growth and pathogen incidence within the greater Stockholm area, Sweden. Water Res. 41 3327–3336.
Leclerc, H., Devriese, L.A. and Mossel, D.A.A. (1996). Taxonomical changes in intestinal (faecal) enterococci and streptococci: Consequences on their use as indicators of faecal contamination in drinking water. J. Appl. Bacteriol., 81(5): 459–466.
Leclerc, H., Mossel, D.A.A., Edberg, S.C., Struijk, C.B. (2001). Advances in the bacteriology of the coliform group: Their suitability as markers of microbial water safety. Ann. Rev. Microbiol., (55):201-234.
Lee, D.-G. and Kim, S.-J. (2003). Bacterial species in biofilm cultivated from the end of the Seoul water distribution system. J. Appl. Microbiol., 95 (2): 317–324.
Lessard, E.J. and Sieburth J. McN., (1983). Survival of natural sewage populations of enteric bacteria in diffusion and batch chambers in the marine environment. Appl. Environ. Microbiol., 45 (3): 950–959.
Léziart, T., Dutheil de la Rochere, P., Cheswick, R., Jarvis, P. and Nocker, A. (2019). Effect of turbidity on water disinfection by chlorination with the emphasis on humic acids and chalk. Environ. Technol., 40(13): 1734-1743.
Locas, A., Barthe, C., Barbeau, B., Carrière, A. and Payment, P. (2007). Virus occurrence in municipal groundwater sources in Quebec, Canada. Can. J. Microbiol., 53 (6): 688–694.
Locas, A., Barthe, C., Margolin, A.B. and Payment, P. (2008). Groundwater microbiological quality in Canadian drinking water municipal wells. Can. J. Microbiol., 54 (6) 472–478.
Maheux, A.F., Picard, F.J., Boissinot, M., Huppé, V., Bissonnette, L., Bernier, J.-.T., Cantin, P., Huletsky, A. and Bergeron, M.G. (2009). Analytical limits of three ß-glucosidase-based commercial culture methods used in environmental microbiology, to detect enterococci. Water Sci. Technol., 60:943–955.
Maheux, A.F., Bissonnette, L., Boissinot, M., Bernier, J.L.T., Huppé, V., Bérubé, E., Boudreau, D.K., Picard, F.J., Huletsky, A. and Bergeron, M.G. (2011). Method for rapid and sensitive detection of Enterococcus sp. and Enterococcus faecalis/faecium cells in potable water samples. Water Res., 45 (6): 2342–2354.
Maheux, A.F., Huppé, V., Bissonnette, L., Boissinot, M., Rodrigue, L., Bérubé, È. and Bergeron, M.G. (2012). Comparative analysis of classical and molecular microbiology methods for the detection of Escherichia coli and Enterococcus spp. in well water. J. Environ. Monit. 14 (11): 2983–2989.
Masters, N., Christie, M., Stratton, H. and Katouli, M. (2015). Viability and stability of Escherichia coli and enterococci populations in fecal samples upon freezing. Can. J. Microbiol., 61 (7): 495–501.
McFeters, G. A., Bissonne, G., Jezeski, J. J., Thomson, C. A. and Stuart, D. G. (1974). Comparative survival of indicator bacteria and enteric pathogens in well water. Appl. Microbiol., 27, 823-829.
MDDELCC, 2016. Bilan de la qualité de l’eau potable au Québec 2010‑2014, 2016, 80 pages, Ministère du Développement durable, de l’Environnement et de la Lutte contre les changements climatiques. Available at http://www.mddelcc.gouv.qc.ca/.
Medeiros, A.W., Blaese Amorim, D., Tavares, M., de Moura, T.M., Franco, A.C., d’Azevedo, P.A., Frazzon, J. and Frazzon, A.P.G. (2017). Enterococcus species diversity in fecal samples of wild marine species as determined by real-time PCR. Can. J. Microbiol., 63 (2): 129–136.
Medema, G.J., Shaw, S., Waite, M., Snozzi, M., Morreau, A. and Grabow, W. (2003). Catchment characteristics and source water quality. In Assessing microbial safety of drinking water: Improving approaches and methods. A. Dufour, M. Snozzi, W. Koster, J. Bartram, E. Ronchi, and L. Fewtrell editors, 111-158. WHO Drinking Water Quality Series, OECD—WHO, Paris, France. London, United Kingdom: IWA Publishing.
Méndez, J., Audicana, A., Cancer, M., Isern, A., Llaneza, J., Moreno, B., Navarro, M., Tarancón, M.L., Valero, F., Ribas, F., Jofre, J. and Lucena, F. (2004). Assessment of drinking water quality using indicator bacteria and bacteriophages. J. Water Health, 2 (3): 201–214.
Mika K.B., Imamura G., Chang C., Conway V., Fernandez G., Griffith J.F., Kampalath R.A., Lee C.M., Lin C.-C., Moreno R., Thompson S., Whitman R.L. and Jay J.A. (2009). Pilot- and bench-scale testing of faecal indicator bacteria survival in marine beach sand near point sources. J. Appl. Microbiol., 107 (1): 72–84.
Moore, D.F., Guzman, J.A. and McGee, C. (2008). Species distribution and antimicrobial resistance of enterococci isolated from surface and ocean water. J. Appl. Microbiol., 105 (4): 1017–1025.
Müller, T., Ulrich, A., Ott, E.-M. and Müller, M. (2001). Identification of plant-associated enterococci. J. Appl. Microbiol., 91 (2): 268–278.
Mundt, J.O., Coggin Jr., J.H. and Johnson, L.F. (1962). Growth of Streptococcus faecalis var. liquefaciens on plants. Appl. Microbiol., 10: 552–555.
Naclerio, G., Petrella, E., Nerone, V., Allocca, V., Vita, P. and Celico, F. (2008). Influence of topsoil of pyroclastic origin on microbial contamination of groundwater in fractured carbonate aquifers. Hydrogeol. J., 16(6): 1057–1064.
NHMRC and NRMMC (2017). Australian drinking water guidelines, Paper 6 National water quality management strategy. National Health and Medical Research Council, National Resource Management Ministerial Council, Commonwealth of Australia, Canberra.
Niño de Guzmán, G.T., Hapeman, C.J., Prabhakara, K., Codling, E.E., Shelton, D.R., Rice, C.P., Hively, W.D., McCarty, G.W., Lang, M.W. and Torrents, A. (2012). Potential pollutant sources in a Choptank River (USA) subwatershed and the influence of land use and watershed characteristics. Science of the Total Environment, 430, pp. 270-279.
Nnane, D.E., Ebdon, J.E. and Taylor, H.D. (2011). Integrated analysis of water quality parameters for cost-effective faecal pollution management in river catchments. Water Res., 45 (6): 2235–2246.
Northern Ireland Water (2016). Drinking water quality annual reports (2010-2014). Northern Ireland Water. Belfast, Northern Ireland. Available from: www.niwater.com
Obiri-Danso, K. and Jones, K. (2000). Intertidal sediments as reservoirs for hippurate negative campylobacters, salmonellae and faecal indicators in three EU recognised bathing waters in North West England. Water Res., 34:519–527.
Ott, E.., Müller, T., Müller, M., Franz, C.M.A.P., Ulrich, A., Gabel, M. and Seyfarth, W. (2001). Population dynamics and antagonistic potential of enterococci colonizing the phyllosphere of grasses. J. Appl. Microbiol., 91: 54–66.
Park, J., Jin, G.-D., Pak, J.I., Won, J. and Kim, E.B. (2017). Development of a rapid identification method for the differentiation of Enterococcus species using a species-specific multiplex PCR based on comparative genomics (2017) Curr. Microbiol., 74 (4): 476–483.
Payment, P. and Locas, A. (2005). Évaluation et contrôle de la qualité virologique des eaux souterraines. Projet: 3331-24-02-01. Institut Armand-Frappier, Institut national de la recherche scientifique. Laval, Québec.
Payment, P. and Locas, A. (2011). Pathogens in water: value and limits of correlation with microbial indicators. Ground Water, 49 (1): 4–11.
Payment, P., Trudel, M. and Plante, R. (1985). Elimination of viruses and indicator bacteria at each step of treatment during preparation of drinking water at seven water treatment plants. Appl. Environ. Microbiol., 49 (6): 1418–1428.
Perez Recuerda, R., Sanchez, J.M. and Borrego, J.J. (1998). The efficiency of different disinfecting agents in inactivating microorganisms detected in natural and treated waters. Tecnol. Agua, 18(177): 16-25.
Peter, A., Mathew, J. and Zacharia, S. (2012). Antibiotic resistant enterococci from drinking water sources. Asian J. Pharm. Clin. Res., 5: 158–160.
Pitkänen, T., Karinen, P., Miettinen, I.T., Lettojärvi, H., Heikkilä, A., Maunula, R., Aula, V., Kuronen, H., Vepsäläinen, A., Nousiainen, L.-L., Pelkonen, S. and Heinonen-Tanski, H. (2011). Microbial contamination of groundwater at small community water supplies in Finland. Ambio, 40 (4): 377–390.
Pitkänen, T., Karinen, P., Miettinen, I.T., Lettojärvi, H., Heikkilä, A., Maunula, R., Aula, V., Kuronen, H., Vepsäläinen, A., Nousiainen, L.-L., Pelkonen, S. and Heinonen-Tanski, H. (2011). Microbial contamination of groundwater at small community water supplies in Finland. Ambio, 40 (4), pp. 377-390.
Pitkänen, T., Ryu, H., Elk, M., Hokajärvi, A.-M., Siponen, S., Vepsäläinen, A., Räsänen, P. and Santo Domingo, J.W. (2017). Detection of fecal bacteria and source tracking identifiers in environmental waters using rRNA-based RT-qPCR and rDNA-based qPCR assays. Environ. Sci. Technol., 47 (23): 13611–13620.
Pote, J., Haller, L., Kottelat, R., Sastre, V., Arpagaus, P. and Wildi, W. (2009). Persistence and growth of faecal culturable bacterial indicators in water column and sediments of Vidy Bay, Lake Geneva, Switzerland. J. Environ. Sci., 21 (1): 62–69.
Poucher, A.M., Devriese, L.A., Hernandez, J.F., and Delattre, J.M. (1991). Enumeration by a miniaturized method of escherichia coli, streptococcus bovis and enterococci as indicators of the origin of faecal pollution of water. J. Appl. Bacteriol., 70: 525–530.
Ramsey, M. Hartke, A. and Huycke, M. (2014). The physiology and metabolism of enterococci. In Enterococci: from commensals to leading causes of drug resistant infection. Gilmore, M.S. et al. (Eds.). Massachusetts Eye and Ear Infirmary, Boston, Massachusetts.
Ran, Q., Badgley, B.D., Dillon, N., Dunny, G.M. and Sadowsky, M.J. (2013). Occurrence, genetic diversity, and persistence of enterococci in a Lake Superior watershed. Appl. Environ. Microbiol., 79: 3067–3075.
Restaino, L., Frampton, E.W., Hemphill, J.B. and Palnikar, P. (1995). Efficacy of ozonated water against various food-related microorganisms. Appl. Environ. Microbiol., 61 (9): 3471-3475.
Rice, E.W., Covert, T.C., Wild, D.K., Berman, D., Johnson, S.A. and Johnson, C.H. (1993). Comparative resistance of Escherichia coli and enterococci to chlorination. J. Environ. Sci. Health - A Environ. Sci. Eng., 28 (1): 89–97.
Risebro, H.L., Breton, L., Aird, H., Hooper, A. and Hunter, P.R. (2012). Contaminated small drinking water supplies and risk of infectious intestinal disease: A prospective cohort study. Plos One 7, (8), art. no. e42762.
Rosen, J.S., Sobrinho, J.A.H. and LeChevallier, M. (2009). Statistical limitations in the usefulness of total coliform data. J. Am. Water Works Assoc., 101: 68–81.
Roser, D.J., Ashbolt, N., Ho, G., Mathew, K., Nair, J., Ryken-Rapp, D. and Toze, S. (2005). Hydrogen sulphide production tests and the detection of groundwater faecal contamination by septic seepage. Water Sci. Technol., 51 (10): 291–300.
Ryu, H., Henson, M., Elk, M., Toledo-Hernandez, C., Griffith, J., Blackwood, D., Noble, R., Gourmelon, M., Glassmeyer, S. and Santo Domingo, J.W. (2013). Development of quantitative PCR assays targeting the 16s rRNA genes of Enterococcus spp. and their application to the identification of Enterococcus species in environmental samples. Appl. Environ. Microbiol., 79 (1): 196–204.
Sánchez Valenzuela, A., Benomar, N., Abriouel, H., Pérez Pulido, R., Martínez Cañamero, M. and Gálvez, A. (2012). Characterization of Enterococcus faecalis and Enterococcus faecium from wild flowers. Antonie van Leeuwenhoek, Int. J. Gen. Mol. Microbiol., 101 (4): 701-711.
Schneeberger, C.L., O'Driscoll, M., Humphrey, C., Henry, K., Deal, N., Seiber, K., Hill, V.R. and Zarate-Bermudez, M. (2015). Fate and transport of enteric microbes from septic systems in a coastal watershed. J. Environ. Health, 77 (9): 22–30.
Sinton, L.W., Davies-Colley, R.J. and Bell, R.G. (1994). Inactivation of enterococci and fecal coliforms from sewage and meatworks effluents in seawater chambers. Appl. Environ. Microbiol., 60 (6): 2040–2048.
Sinton, L.W., Hall, C.H., Lynch, P.A. and Davies-Colley, R.J. (2002). Sunlight inactivation of fecal indicator bacteria and bacteriophages from waste stabilization pond effluent in fresh and saline waters. Appl. Environ. Microbiol., 68(3): 1122–1131.
Sinton, L.W. and Donnison, A.M. (1994). Characterisation of faecal streptococci from some New Zealand effluents and receiving waters. N. Z. J. Mar. Freshwater Res., 28 (2): 145–158.
Smeets, P., Rietveld, L., Hijnen, W., Medema, G. and Stenstrom, T-A. (2006). Efficacy of water treatment processes. In MicroRisk - Microbiological risk assessment: a scientific basis for managing drinking water safety from source to tap. April.
Speight, V.L., Kalsbeek, W.D. and DiGiano, F.A. (2004). Randomized stratified sampling methodology for water quality in distribution systems. J. Water Resour. Plann. Manag., 130: 330–338.
Splichalova, P., Svec, P., Ghosh, A., Zurek, L., Oravcova, V., Radimersky, T., Bohus, M. and Literak, I. (2015). Prevalence, diversity and characterization of enterococci from three coraciiform birds. Antonie van Leeuwenhoek, Int. J. Gen. Mol. Microbiol., 107 (5): 1281–1289.
Staley, C., Dunny, G.M. and Sadowsky, M.J. (2014). Environmental and animal-associated enterococci. Adv. Appl. Microbiol., 87: 147–186.
Stange, C., Sidhu, J.P.S., Toze, S. and Tiehm, A. (2019). Comparative removal of antibiotic resistance genes during chlorination, ozonation, and UV treatment. Int. J. Hyg. Environ. Health, 222(3): 541-548.
Suzuki, Y., Kanda, N. and Furukawa, T. (2012). Abundance of Enterococcus species, Enterococcus faecalis and Enterococcus faecium, essential indicators of fecal pollution, in river water. J. Environ. Sci. Health, Part A: Toxic/Hazard. Subst. Environ. Eng., 47 (11): 1500–1505.
Svec, P. and Devriese, L.A. (2009). Genus Enterococcus. In Bergey's manual of systematic bacteriology, 2nd edition. De Vos, P., Garrity, G.M., Jones, D., Krieg, N.R., Ludwig, W., Rainey, F.A., Schleifer, K.H. and Whitman, W.B. (Eds.). Springer. New York, New York.
Tavakoli, A., Yazdani, R., Shahmansouri, M.R. and Isfahani, B.N. (2005). Chlorine residual efficiency in inactivating bacteria from secondary contamination in Isfahan, 2002. East. Mediterr. Health J., 11(3): 425-434.
Tendolkar, P.M., Baghdayan, A.S. and Shankar, N. (2003). Pathogenic enterococci: New developments in the 21st century. Cell. Mol. Life Sci., 60 (12): 2622–2636.
U.S. EPA (2006a). 40 CFR parts 9, 141 and 142. National primary drinking water regulations: Ground water rule. Final rule. Fed. Regist., 71(216): 65573–65660.
U.S. EPA (2006b). Method 1600: Enterococci in water by membrane filtration using membrane-Enterococcus Indoxyl-$-D-Glucoside Agar (mEI). Office of Water, United States Environmental Protection Agency. Washington, DC. June. EPA-821-R-06-009.
U.S. EPA (2015a). Method 1609.1. Enterococci in water by TaqMan® quantitative polymerase chain reaction (qPCR) with internal amplification control (IAC) assay. Office of Water, United States Environmental Protection Agency. Washington, DC. April. EPA-820-R-15-099.
U.S. EPA (2015b). Method 1611.1. Enterococci in water by TaqMan® quantitative polymerase chain reaction (qPCR) assay. Office of Water, United States Environmental Protection Agency. Washington, DC. April. EPA-820-R-15-008
U.S. EPA (2017). Analytical methods approved for compliance monitoring under the groundwater rule. Office of Water, U.S. Environmental Protection Agency, Washington, DC. EPA 821-F-17-004.
van der Kooij, D. and van der Wielen, P.W.J.J. (2014). Chapter 15 – Research needs. In Microbial growth in drinking-water supplies: Problems, causes, control and research needs. IWA Publishing, London, UK. Pp. 423-443
van Lieverloo, J.H.M., Mesman, G.A.M., Bakker, G.L., Baggelaar, P,K., Hamed, A. and Medema, G. (2007). Probability of detecting and quantifying fecal contamination of drinking water by periodically sampling for E. coli: a simulation model study. Water Res., 41: 4299–4308.
Wade, T.J., Calderon, R.L., Sams, E., Beach, M., Brenner, K.P., Williams, A.H. and Dufour, A.P. (2006). Rapidly measured indicators of recreational water quality are predictive of swimming-associated gastrointestinal illness. Environ. Health Perspect., 114 (1): 24–28.
Wade, T.J., Sams, E., Brenner, K.P., Haugland, R., Chern, E., Beach, M., Wymer, L., Rankin, C.C., Love, D., Li, Q., Noble, R. and Dufour, A.P. (2010). Rapidly measured indicators of recreational water quality and swimming-associated illness at marine beaches: A prospective cohort study. Environ. Health (London, U.K.), 9 (1), art. no. 66.
Wedgworth, J.C., Brown, J., Olson, J.B., Johnson, P., Elliott, M., Grammer, P. and Stauber, C.E. (2015). Temporal heterogeneity of water quality in rural Alabama water supplies. J. Am. Water Works Assoc., 107 (8): E401–E415.
Weigand, M.R., Ashbolt, N.J., Konstantinidis, K.T. and Santo Domingo, J.W. (2014). Genome sequencing reveals the environmental origin of enterococci and potential biomarkers for water quality monitoring. Env. Sci. Technol., 48 (7):3707-3714.
Whitman, R.L., Shively, D.A., Pawlik, H., Nevers, M.B. and Byappanahalli, M.N. (2003). Occurrence of Escherichia coli and enterococci in Cladophora (Chlorophyta) in nearshore water and beach sand of Lake Michigan. Appl. Environ. Microbiol., 69: 4714–4719.
WHO (1971). International standards for drinking-water, 3rd edition. World Health Organization, Geneva, Switzerland.
WHO (1976). Surveillance of drinking-water quality. World Health Organization, Geneva, Switzerland. (WHO Monograph Series No. 63).
WHO (1997). Guidelines for drinking-water quality (Vol 2 and 3). World Health Organization. Geneva, Switzerland.
WHO (2004). Guidelines for drinking-water quality. Third Edition. World Health Organization. Geneva, Switzerland.
WHO (2005). Water safety plans - Managing drinking-water quality from catchment to consumer. World Health Organization, Geneva, Switzerland.
WHO (2017). Guidelines for drinking-water quality. Fourth Edition. World Health Organization. Geneva, Switzerland.
WHO and OECD (2003). Assessing Microbial Safety of Drinking Water – Improving approaches and methods. World Health Organization and Organisation for Economic Co-operation and Development. IWA Publishing, London U.K.. pp 1-295.
Wiedenmann, A., Braun, M. and Botzenhart, K. (1997). Evaluation of the disinfection potential of low chlorine concentrations in tap water using immobilised Enterococcus Faecium in a continuous flow device. Water Sci. Technol., 35 (11-12): 77-80.
Wilkes, G., Edge, T., Gannon, V., Jokinen, C., Lyautey, E., Medeiros, D., Neumann, N., Ruecker, N., Topp, E. and Lapen, D.R. (2009). Seasonal relationships among indicator bacteria, pathogenic bacteria, Cryptosporidium oocysts, Giardia cysts, and hydrological indices for surface waters within an agricultural landscape. Water Res., 43 (8): 2209–2223.
Wu, J., Long, S.C., Das, D. and Dorner, S.M. (2011). Are microbial indicators and pathogens correlated? A statistical analysis of 40 years of research. J. Water Health, 9 (2): 265–278.
Yard, E.E., Murphy, M.W., Schneeberger, C., Narayanan, J., Hoo, E., Freiman, A., Lewis, L.S. and Hill, V.R. (2014). Microbial and chemical contamination during and after flooding in the Ohio River-Kentucky, 2011. J. Environ. Sci. Heal. A., 49 (11), pp. 1236-1243.
C.2 List of acronyms
- ANSI
- American National Standards Institute
- CFU
- colony forming units
- DNA
- deoxyribonucleic acid
- EU
- European Union
- ISO
- International Organization for Standardization
- MF
- membrane filtration
- MTF
- multiple tube fermentation
- NSF
- NSF International
- PCR
- polymerase chain reaction
- qPCR
- quantitative polymerase chain reaction
- RNA
- ribonucleic acid
- U.S. EPA
- United States Environmental Protection Agency
- UV
- ultraviolet
- VBNC
- viable but non-culturable
- WHO
- World Health Organization
Page details
- Date modified: