Guidelines for Canadian Drinking Water Quality Guideline Technical Document - Uranium
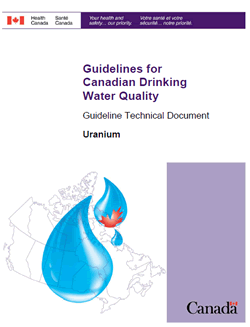
Download the alternative format
(PDF format, 728 KB, 87 pages)
May 2019
ISBN: 978-0-660-29988-4
Cat.: H144-13/12-2019E-PDF
HC Pub.: 180870
Organization: Health Canada
Table of Contents
Part I. Overview and Application
Part II. Science and Technical Considerations
- 4.0 Identity, use and sources in the environment
- 5.0 Exposure
- 6.0 Analytical methods
- 7.0 Treatment technology
- 7.1 Uranium occurrence as a function of water quality and chemistry
- 7.2 Municipal scale
- 7.3 Residuals management
- 7.4 Uranium in the distribution system
- 7.5 Residential scale
- 8.0 Kinetics and metabolism
- 9.0 Health effects
- 10.0 Classification and assessment
- 11.0 Rationale
- 12.0 References
Part I. Overview and Application
1.0 Guideline
A maximum acceptable concentration (MAC) of 0.02 mg/L (20 µg/L) is established for total natural uranium in drinking water.
The guideline is based on the chemical toxicity of naturally-occurring uranium.
2.0 Executive summary
Uranium is widespread in nature and has been identified in many different minerals. It exists in several chemical oxidation states. Although natural uranium is a weakly radioactive substance, the principal health effects associated with natural uranium are due to its chemical toxicity. The maximum acceptable concentration is established based on the chemical toxicity of uranium, and the focus of this document is limited to uranium's chemical properties. Radiological forms and/or radioactive isotopes of uranium are addressed in a separate document (Health Canada, 2009).
This guideline technical document reviews and assesses all identified health risks associated with natural uranium in drinking water. It incorporates new studies and approaches and takes into consideration the availability of appropriate treatment technology. Based on this review, the drinking water guideline for total uranium re-establishes the maximum acceptable concentration (MAC) of 0.02 mg/L (20 µg/L).
2.1 Health effects
With respect to chemical toxicity, international organizations agree that there is insufficient evidence to conclude that oral exposure to natural uranium will cause cancer in humans or animals. Studies in humans suggest that chronic exposure to uranium in drinking water may affect the kidneys. Consequently, the MAC of 0.02 mg/L has been developed based on studies of kidney effects in rats. Exposure through inhalation and skin contact is not considered to be significant.
2.2 Exposure
Canadians are primarily exposed to uranium through drinking water and food. Uranium may be found in water in the environment as a result of weathering and leaching from natural deposits, fallout from volcanic eruptions, its release in mill tailings, emissions from the nuclear industry, the use of phosphate fertilizers and the combustion of coal and other fuels. Levels of uranium in drinking water vary greatly across Canada, depending mainly on geological formations and to a lesser extent on human activities in the watershed.
2.3 Analysis and treatment
There are several approved analytical methods available to measure total uranium in drinking water at levels well below the MAC.
The concentration of naturally-occurring uranium in groundwater depends on factors such as pH, redox potential and the presence of dissolved oxygen and complexing agents. The chemical speciation of uranium in the water supply is important to ensure its successful removal during treatment process. Most groundwaters contain soluble uranium complexes.
At the municipal level, the best available technologies for the treatment of total uranium are coagulation/filtration, lime softening, ion exchange and reverse osmosis. In addition, management strategies for uranium include practices such as switching to a new source, blending and interconnecting with another water system.
At the residential level, there are no certified residential treatment devices for the reduction of uranium from drinking water. However, drinking water treatment technologies able to effectively remove uranium include ion exchange and reverse osmosis. It is important to note that reverse osmosis systems should be installed only at the point of use, as the treated water may be corrosive to internal plumbing components.
2.4 International Considerations
Drinking water quality guidelines, standards and/or guidance established by foreign governments or international agencies may vary due to the science available at the time of assessment, as well as the utilization of different policies and approaches, such as the choice of key study, and the use of different consumption rates, body weights and allocation factors.
The maximum contaminant level of U.S. Environmental Protection Agency and the provisional guideline value of World Health Organization are both 30 µg/L for uranium. The Australian National Health and Medical Research Council has established a guideline 17 µg/L. As of 2018, the European Union has not established a drinking water value for natural uranium.
3.0 Application of the guideline
Note: Specific guidance related to the implementation of drinking water guidelines should be obtained from the appropriate drinking water authority in the affected jurisdiction.
Although natural uranium is a weakly radioactive substance, the principal health effects associated with natural uranium are due to its chemical toxicity. The focus of this document is limited to uranium's chemical properties and the MAC for natural uranium is based on chemical uranium. Radiological forms and/or radioactive isotopes of uranium are addressed in a separate document (Health Canada, 2009).
For drinking water supplies that occasionally experience short-term exceedances of uranium above the guideline values, it is suggested that a plan be developed and implemented to address these situations. For more significant, long-term exceedances that cannot be addressed through treatment, it is suggested that alternative sources of drinking water be considered.
Since other radionuclides can co-occur with uranium, special precautions may be required when waste streams (residuals) are treated, stored, disposed of or transported. The appropriate authorities should be consulted to ensure that the disposal of liquid and solid waste residuals from the treatment of drinking water meet applicable regulations. At the residential level, devices are generally not expected to contain enough radioactivity to warrant special precautions by homeowners.
3.1 Monitoring
3.1.1 Source characterization
Source water should be characterised to determine whether uranium is present. Given the ubiquitous nature of nitrate in groundwater and its strong correlation with uranium mobilization, groundwater should be tested for both uranium and nitrate. For groundwaters with a nitrate concentration above or near its MAC, more frequent monitoring of the source water for uranium should be considered. Authorities may reduce monitoring when it has been demonstrated that uranium is present at concentrations well below the MAC in the source water, that there is no reason to expect that the uranium concentration will vary greatly with time or that appropriate treatment is in place. Since radionuclides such as radium may co-occur with uranium, authorities may want to consider gross alpha activity screening when uranium is present. The guideline technical document on radiological parameters should be consulted for further information.
Utilities practicing control options for addressing uranium concentration in source water used for drinking should assess the water quality of the new sources to ensure that it does not interfere with the existing treatment processes, impact the distribution system, and cause other water quality issues.
3.1.2 Treatment and processes monitoring
Utilities that treat their water to remove uranium need to conduct frequent monitoring of treated water in order to make necessary process adjustments and to ensure that treatment processes are effectively removing uranium and other co-contaminants below their respective MACs.
Utilities using conventional coagulation/filtration and lime softening for uranium removal should conduct operational monitoring of pH. Water treated by lime softening may require re-carbonation to reduce pH, as well as the addition of corrosion-inhibiting chemicals to protect the distribution system.
Utilities using ion exchange to remove uranium should monitor the pH of the treated water, particularly at the beginning of an ion exchange treatment run. Freshly regenerated anion exchange resins reduce the pH and total alkalinity, and the pH may need to be increased during this period to control corrosion. Another operational consideration is the chromatographic peaking of other anions such as arsenic. Since uranium complexes have a high affinity for the anion exchange resins, less preferred anion may be released in the treated water.
Reverse osmosis and ion exchange are often operated with a bypass blending a portion of the influent flow with the treated water to obtain desired water quality. In such cases, it is important to monitor blended water quality to determine the final uranium concentration.
3.1.3 Compliance monitoring
When uranium is present in source water, it is recommended that compliance monitoring be conducted annually, to confirm that the MAC is not exceeded. Samples should be collected prior to distribution (typically at the entry point to the distribution system) and analysed by an accredited laboratory.
3.1.4 Distribution system
As with other inorganics, uranium can be accumulated in distribution systems and later released. Since the stability of metals accumulated in the scales is unpredictable, it is difficult to establish a monitoring program for uranium in distribution systems. Factors that influence uranium accumulation and release in distribution system (co-occurrence of iron and manganese in the deposits, changes to pH conditions and hydraulic disturbances) could be used as indicators of when and where to monitor for uranium releases in distribution systems. When water quality or hydraulic disruptions occur in the system, the release of uranium and other contaminants may be indicated by the presence of discoloured water or increased turbidity resulting from the release of deposits or scales present on pipe wall. When this occurs, monitoring for uranium and other constituents should be conducted. Monitoring for uranium should be done in conjunction with other metals that can co-occur in the distribution system (e.g., iron, manganese, arsenic, lead). However, the absence of discoloured water should not be interpreted as the absence of metal release.
Part II. Science and Technical Considerations
4.0 Identity, use and sources in the environment
4.1 Identity
Uranium (CAS Registry No. 7440-61-1) is a dense, silvery-white, weakly radioactive metal that rapidly oxidizes when exposed to air (CCME, 2007; Haynes, 2014). Natural uranium never occurs in its elemental state but is always combined with other elements (McDiarmid et al., 2012). It has been identified in 150 different minerals and is widespread in nature, occurring in granites, metamorphic rocks, lignite, monazite sands, phosphate and various other mineral deposits (Cothern and Lappenbusch, 1983; McDiarmid et al., 2012; Haynes, 2014). Uranium exists in the +2, +3, +4, +5 or +6 oxidation states although the tetravalent and hexavalent states are the most common (CCME, 2007; Haynes, 2014). The hexavalent state is also the most stable form in solution and the most biologically relevant form (Cothern and Lappenbusch, 1983; LaTouche et al., 1987).
Natural uranium is a mixture of three radioisotopes 238U, 235U and 234U, in concentrations of 99.2745%, 0.720% and 0.0055%, respectively (Haynes, 2014). These three isotopes have the same number of protons and exhibit the same chemical toxicity. Despite all naturally occurring isotopes of uranium being considered weakly radioactive, the health effects of natural uranium are due to its chemical toxicity (Kathren and Burklin, 2008; ATSDR, 2013).
4.2 Use
Uranium is mined primarily for use as fuel in nuclear power stations (WHO, 2011). Canada is the second largest producer of uranium in the world, accounting for 15% of global production in 2012. The Canadian uranium industry also mills, refines and converts uranium into uranium dioxide and uranium hexafluoride, and produces fuel bundles. Canada's only uranium refinery is in Blind River, Ontario (Natural Resources Canada, 2014). Further processing takes place at Port Hope, Ontario. Fuel pellets and/or bundles are manufactured in Port Hope, Peterborough and Toronto (Canadian Nuclear Safety Commission, 2012). Canada has no uranium enrichment plants and does not produce depleted uranium (DU)(Canadian Nuclear Safety Commission, 2009).
4.3 Sources and fate
Depending on the underlying rock formation, some geographical areas naturally contain high levels of uranium (CCME, 2011). Canada has large uranium deposits with known resources of 490,000 tonnes and identified uranium ore bodies in: British Columbia (Blizzard and Prairie Flats); Athabasca region of northern Saskatchewan (Midwest Deposit); Ontario (Bancroft and South March); Labrador (Kaipokok); and in the Northwest Territories (Lineament Lake) (CCME, 2007; Natural Resources Canada, 2014). Mill effluents, produced when uranium is mined, can release selenium and molybdenum into the environment (CNSC, 2014).
Uranium is present in water supplies as a result of weathering and leaching from natural deposits, fallout from volcanic eruptions, its release in mill tailings, emissions from the nuclear industry and the combustion of coal and other fuels (Dreesen et al., 1982; Cothern and Lappenbusch, 1983; Essein et al., 1985; Tadmor, 1986). Phosphate fertilizers, which may contain uranium at concentrations as high as 150 mg/kg, may also contribute to the uranium content of groundwater while nitrate fertilizers may mobilize existing stores of uranium (Spalding and Sackett, 1972; Jacques et al., 2008; Nolan and Weber, 2015).
The mobility of uranium in the environment is highly dependent on the chemical and physical form of the uranium, its oxidation state, its pH, its solubility, the presence of inorganic/organic ligands, and its adsorption and desorption on surface water sediments (EFSA, 2009; ATSDR, 2013). Oxidized forms of uranium are relatively soluble and can be leached from the rocks to migrate in the environment (ATSDR, 2013). In oxic natural waters, uranium is present mainly in the U(VI) state (oxidized) either as the free uranyl ion, UO22+ or complexed to a ligand to form soluble complexes with carbonate, bicarbonate, fluorides or sulphate ions, enhancing its mobility. It also complexes with phosphates to form insoluble precipitates (Cothern and Lappenbusch, 1983). Tetravalent uranium is present in strongly reducing environments (e.g., anoxic waters) where it interacts with hydroxides, hydrated fluorides and phosphates to form insoluble compounds which precipitate out of solution (CCME, 2007; ATSDR, 2013). Under oxidizing conditions, the tetravalent state is readily oxidized to the hexavalent state (EFSA, 2009).
Studies indicated that the presence of nitrate and ferrous iron [Fe (II)] in groundwater containing U(IV)sediments leads to the remobilization of U(IV) through various oxidation reactions (Senko et al., 2002; Istok et al., 2002). The oxidation of Fe(II) by microorganisms (Straub et al. 2001; Lack et al. 2002) or by nitrite that accumulates under dissimilatory nitrate reduction (i.e., denitrification) conditions (Senko et al. 2002, 2005) can lead to the presence of Fe(III). Nevin and Lovley (2000) and Senko et al. (2002) postulated that the nitrate-dependent U(IV) oxidation actually proceeds via the oxidation of U(IV) to U(VI) by Fe(III). These processes have been shown to occur at approximately neutral pH and the generated Fe(III) was found to be extremely reactive with U(IV) (Senko et al. 2005).
Nitrate is a common groundwater contaminant which is known to alter uranium solubility by oxidation of the U(IV) to U(VI). Abiotic and biotic nitrate reduction reactions produce nitrite and nitrous oxide which are also capable of oxidizing U(IV) to U(VI) (Senko et al., 2002, 2005). Nolan and Weber (2015) investigated co-occurrence of nitrate and uranium in two major U.S. aquifers (High Plains and Central Valley; n=53,352 wells) and established a link between uranium and nitrate concentrations. The authors reported that areas with a nitrate concentration of 7.0 mg/L or higher were strongly correlated with uranium concentrations equal or greater than 20 µg/L. The study indicated that the nitrate concentration was strongly correlated to the uranium concentration and concluded that shallow groundwater wells were more susceptible to co-contamination than deep wells.
The amount of uranium mobilized into ground and surface water can change over time, increasing with changing oxidizing conditions or alkaline conditions (e.g., increased calcium from the application of road salts), and from excessive drawdown caused by groundwater pumping or seasonal fluctuations (Brown et al., 2007; Keith et al., 2007; Jurgens et al., 2010; Drage and Kennedy, 2013; Bexfield and Jurgens, 2014). Changes in environmental conditions (e.g., dry periods followed by rain) can also alter uranium concentrations in water bodies (Gilson et al., 2015). In most waters, sediments act as a sink for uranium and the uranium concentrations in sediments and suspended solids are several orders of magnitude higher than in surrounding water (ATSDR, 2013). A strong correlation between uranium and bicarbonate concentrations suggested that uranium is leached from shallow sediments by water containing bicarbonate ions (Jurgens et al., 2010).
5.0 Exposure
Food and water represent the main sources of exposure to uranium but the contribution from these sources can be highly variable. In the case of Canadian drinking water, uranium content can vary greatly depending on geological formations and anthropogenic activities surrounding the source water. Allocating 50% source contribution to drinking water is deemed appropriate given only two major sources of exposure have been identified (Krishnan and Carrier, 2013) and the data for these exposure sources is highly variable. It is also supported by both the European Food Safety Authority (EFSA, 2009) and the National Council on Radiation Protection and Measurements (NCRPM, 1984) who both have estimated that drinking water accounts for 50% of uranium intake by humans. California EPA (OEHHA, 2001) and Anke et al. (2009) attributed 40% and 41% of uranium intake to drinking water and drinking water/beverages respectively.
5.1 Water
Uranium levels in drinking water can vary greatly depending on geological formations surrounding the source water, on the presence of factors affecting uranium mobilization (Sec. 4.3) and on the proximity of source water to uranium facilities. In Canada, data indicate that uranium is found across the country. Based on the original data provided by the provinces and territories, the mean uranium content for drinking water was calculated as 4.35 µg/L across the country with an adult drinking water intake value of 0.09 µg/kg bw per day.
The average uranium content for 3221 raw samples from both surface and groundwater sources in Newfoundland was 1.5 µg/L. The range of values was 0.1 to 20 µg/L (Newfoundland and Labrador Department of Environment and Conservation, 2015).
Samples collected over approximately 10 years in Nova Scotia gave an average of 2.27 µg/L of uranium in both raw and treated water from surface and groundwater sources. Concentrations ranged from <0.1 to 19 µgL with 586 out of 1055 samples below the detection limit of <0.1 µg L (Nova Scotia Environment, 2015).
In New Brunswick, 3745 samples of raw and treated water were tested over a 10 year period and averaged 0.11 µg/L of uranium. Values ranged from <0.05 to165 µg/L. Only four values exceeded 30 µg/L; these were all for raw water samples (New Brunswick Department of Health, 2015).
Nine years of data from Quebec had an average uranium concentration of 6.7 µg/L. Of the 28,214 samples tested, 3294 were above the detection limit of 1 µg/L. The maximum value recorded was 360 µg/L. One hundred and twenty eight samples were above the provincial limit of 20 µg/L for uranium (Ministère du Développement durable, de l'Environnement et de la Lutte contre les changements climatiques du Québec, 2015).
For Ontario, a total of 11528 samples of raw and treated water, taken over an eleven year period, were analyzed for uranium and averaged 0.6 µg/L. Values ranged from undetectable to 17.5 µg/L (Ontario Ministry of the Environment and Climate Change, 2015).
Concentrations of total uranium in Manitoba surface waters are naturally high. Between 2000 and 2016, approximately 8,315 surface water samples (e.g., lakes, rivers, streams, creeks, wetlands) were collected and analyzed for total uranium by Manitoba Sustainable Development. In total, 4,267 out of the 8,315 (51%) exceeded 1 μg/L. In addition, there were some rivers and creeks that exceeded the 14 ug/L drinking water quality guideline (e.g., Sturgeon Creek, Boyne River, Souris River, Wood Lake); although this was rare. Approximately 14% of observations (i.e. 1,215 out of 8,315 samples) were below a detection limit of 0.0001 mg/L (or 0.1 μg/L) (Manitoba Sustainable Development, 2018).
Saskatchewan had an average uranium concentration of 5.5 µg/L, representing 10 years of data consisting of 3910 of both treated and raw water samples. The values ranged from 0.0034 to 183 µg/L. Two hundred and fifty three samples were above 20 µg/L. These high samples had an average uranium concentration of 36.9 µg/L (Saskatchewan Water Security Agency, 2015).
Data collected over the last 16 years in Alberta showed an average uranium concentration of 0.8 µg/L and a maximum of 11 µg/L in raw water samples (n=461), while treated water samples (n=1500) had an average concentration of 0.7 µg/L and a maximum of 34 µg/L (Alberta Environment and Parks, 2015).
A combined average uranium concentration of 11.4 µg/L was calculated for British Columbia based on 11 samples of raw and treated water. Values ranged from 0.035 to 29 µg/L (British Columbia Ministry of Health, 2015). Uranium concentrations in groundwater in the Lower Mainland and Okanagan/Kamloops areas are available for Osoyoos (83.6 µg/L), Kelowna (33.6 µg/L and 193.8 µg/L), Surrey (35 µg/L), Kamloops (37 µg/L and 50 µg/L), Dawson Creek (160.1 µg/L) (British Columbia Ministry of Environment and Climate Change Strategy, 2017). An average uranium concentration of 13.32 µg/L for both raw and treated water was available for the Yukon for 2011-2015. A total of 91 samples were collected from 26 locations with a range of <0.1 to 246 µg/L. Eight values were greater than 20 µg/L and were all from the same location for untreated water (Yukon Environmental Health Services, 2015).
Twenty samples (source: 1 glacier, 19 surface water) of raw water collected in Nunavut had a concentration range of 0.010 to 2.19 µg/L of uranium with an average concentration of 0.29 µg/L (Nunavut Department of Health, 2015).
Data from the Canadian National Drinking Water Survey (Health Canada, 2015d) averaged to 0.52 μg/L (n=646) and ranged from below the detection limit of 0.1 to 32 μg/L.
5.2 Food
The content of uranium in food is highly variable and dependent on the concentration of uranium in agricultural soil, the preferential uptake of uranium by certain plants and plant parts, and plant age. Leafy plants grown in uranium-rich soil accumulated more uranium than did tubers, thick stalks, fruits or grains while uranium content decreased with increasing plant age (Anke et al., 2009). Animal foodstuffs are generally poor in uranium except for marine fish and shellfish which can contain high levels (Shiraishi et al., 2000). Depending on an individual's diet and the source of food items eaten (e.g., local), food can be a significant source of uranium consumed accounting for an estimated 40 to 60% of daily uranium intake (OEHHA, 2001; Anke et al., 2009; EFSA, 2009).
A Total Diet Study (TDS) was conducted by Health Canada from 1993 to 2012. It used typical consumption rates for various foods (210 individual food items) to estimate a range of average daily uranium intake in different age-sex groups of Canadians. The study was conducted in Ottawa, St. John's, Vancouver, Montreal, Winnipeg, Toronto and Halifax. Uranium concentrations for food items sampled (n=1676) ranged from below detection limits (<0.008 ng/g) to 314 ng/g, with an average concentration of 0.704 ng/g. Most foods (97% of samples) had uranium concentrations of <10 ng/g (Health Canada, 2015a).
In the same TDS, concentrations of uranium in milk (including 1%, 2%, skim, and whole) were all less than 0. 07 ng/g (average of 0.03 ng/g) while concentrations in reconstituted baby formulae ranged from 0.39 to 4.3 ng/g for milk based formulae (average of 2.70 ng/g) and from 3.9 to 8.2 ng/g for soy-based formulae (average of 6.30 ng/g). Fruits, most grains, nuts, sugar, honey, meats (except processed) and freshwater fish were low in uranium, with average values below 0.40 ng/g. Marine fish (average of 1.46 ng/g), shellfish (average of 7.21 ng/g), most vegetables tested (average of 3.62 ng/g; range 0.12 to 8.17 ng/g), and herbs and spices (average of 13.22 ng/g) had higher uranium contents. The products with the highest uranium concentrations were baking powder (average of 184 ng/g) and chewing gum (average of 120 ng/g; maximum 314 ng/g) (Health Canada, 2015a).
The range of average dietary intakes of uranium across cities was 0.06 to 0.09 µg/kg bw per day with an overall average of 0.07 µg/kg bw per day across sexes and age groups (Health Canada, 2011). Some age differences were reported. Infants up to 6 months of age had intake values of 0.22 µg /kg bw per day. Intake levels were 0.12, 0.09 and 0.06 µg/kg bw per day for children 7 months to 4 years of age, 5 to 11 years of age and 12 to 19 years of age, respectively. Adults (≥20 years) had uranium intake levels of 0.08 µg/kg bw per day (Health Canada, 2011).
No Canadian data for uranium content in breast milk were located, although data from European studies were available. A study conducted in Poland, Germany and the Czech Republic found mean uranium concentrations of 0.03 µg/L in breast milk from samples taken daily over 2 to 8 weeks (n=19) (Wappelhorst et al., 2002). Maternal dietary uranium intake via food consumption was also measured giving a mean of 0.03 µg/kg bw per day. A study conducted in Sweden found a mean uranium content of 0.42 µg/L from breast milk sampled twice daily by mothers (n=60) over one week. The samples for each mother were pooled. No data relating to maternal dietary uranium intake were recorded (Ljung Bjorklund et al., 2012).
5.3 Air
Data for ambient atmospheric uranium concentrations in Canada were available from Environment Canada. Close to ten thousand air samples (n=9703) were taken between 2010 and 2015, ranging in values from undetectable levels to 0.081 ng/m3 with an overall average concentration in air of 0.002 ng/m3 across Canada. Seventeen of the 26 values above 0.020 ng/m3 were collected in Ontario. The average air concentrations of uranium by province/territory for which data were available are: 0.001 ng/m3 for British Columbia, New Brunswick and Nova Scotia; 0.002 ng/m3 for Alberta, Ontario and Quebec; and 0.03 ng/m3 for Manitoba and the Northwest Territories (Health Canada, 2015b).
Health Canada has been monitoring uranium exposure in and around Port Hope's (Ontario) two uranium facilities for more than 30 years (Canadian Nuclear Safety Commission, 2009). The most recent concentrations in ambient air taken in 2011 ranged from 0.28 to 5 ng/m3 (Canadian Nuclear Safety Commission, 2009; Lane et al., 2011) and show concentrations are decreasing with time when compared to historical data (< 0.06 to 200 ng/m3) (Tracy and Meyerhof, 1987; Ahier and Tracy, 1997). More recent data provided by Cameco in its 2014 annual report gave an annual average ambient air concentration of uranium in Port Hope of 2 ng/m3 (Cameco, 2015).
Based on these low concentrations of uranium in ambient air, intake from air is negligible compared to that from other media.
5.4 Consumer products
No specific, current consumer products were identified as a source of uranium exposure for the general Canadian population. Historically, uranium was added as a colourant to glass and ceramic glazes used for food and glassware items such as glazed ceramics and Vaseline glass. Uranium can leach from such antiques in amounts ranging from 30 µg/L (Vaseline glass) to 300,000 µg/L (glazed ceramics) following contact with foodstuff or beverages (Landa and Councell, 1992; Sheets and Thompson, 1995).
5.5 Soil
Since uranium is naturally occurring, its distribution across the country can vary greatly depending on rock type (CCME, 2007). In general, the uranium content of rock varies from 0.5 to 4.7 µg/g in igneous rocks like basalts and granites to 2.0 µg/g in carbonate rocks and up to 3.7 µg/g in sedimentary rocks such as shale (NCRPM, 1984). The average background level of uranium in soil is around 2 µg/g (NCRPM, 1984; CCME, 2007).
Data specific to uranium concentrations in Canadian soils were identified for British Columbia (BC), Saskatchewan, Manitoba, Ontario, New Brunswick and Quebec. These ranged from 0.4 to 15 µg/g (van Netten and Morley, 1982; Gordon, 1992; OMEE, 1993; Gizyn, 1994; Lin et al., 1996; Pilgrim and Schroeder, 1997; Thomas, 2000; Rasmussen et al., 2001; CCME, 2007; Canadian Nuclear Safety Commission, 2014).
5.6 Multi-route exposure through drinking water
Given the physicochemical properties of uranium, a multi-route exposure assessment, as outlined by Krishnan and Carrier (2008), could not be performed. Exposure to uranium by inhalation during showering is not expected to occur since uranium is not known to form any volatile species under such conditions (Clark et al., 2006). Additionally, only small particles of less than 2.5 microns are deposited in the lungs while large uranium particles (e.g., in water droplets) are effectively cleared by mucocilliary action (Patocka, 2014).
Uranium penetration through intact skin in humans has not been well documented (ATSDR, 2013). A single patch test study using up to 25% uranyl acetate in petrolatum applied to the skin of veterans previously exposed to depleted uranium indicated that uranium could potentially be absorbed across the skin although the high dose of uranium used and the presence of imbedded depleted uranium make the findings difficult to apply to environmental exposure (Shvartsbeyn et al., 2011). Limited animal data show dermal absorption can occur in animals following application of high amounts of soluble uranium compounds (de Rey et al., 1983). LD50 (dermal) values for uranyl nitrate hexahydrate, measured as uranium, ranged from 59 mg/kg bw for rabbits to 7600 mg/kg bw for mice (Orcutt, 1949). Only animals that died showed renal damage. The sites of application showed superficial coagulation necrosis, suggesting skin damage caused by the chemical may have increased absorption. Additionally, animals were not prevented from licking the site of application which may have contributed to the observed toxicity. de Rey et al. (1983) suggested that the large amount of uranium absorbed as uranyl nitrate might be caused by NO2 anion interacting with membrane lipoprotein complexes. The relevance of these findings in humans is unclear as the concentrations of uranium applied to these animals were extremely high and it is unlikely that humans would be exposed to such high levels from the environment.
Thus, at uranium levels typically reported in Canadian drinking water supplies, exposure via the dermal and inhalation routes is not expected to be significant during showering and bathing.
5.7 Blood and urine levels in the Canadian population
Urinary uranium concentrations are considered the best method for biological monitoring of environmentally and occupationally exposed populations (McDiarmid et al., 2012; ATSDR, 2013) and have been used in a number of epidemiological studies (Harduin et al., 1994; Limson Zamora et al., 1998; Kurttio et al., 2002; Al-Jundi et al., 2004) however, background values for unexposed populations are scant. Uranium levels in urine are sometimes normalized based on urinary creatinine concentrations, although this practice has recently come under debate (Hoet et al., 2015). Blood, hair and nails have also been used for biomonitoring. There is discussion as to whether the use of hair (Karpas et al., 2005; Muikku et al., 2009; Israelsson and Pettersson, 2014; Joksić and Katz, 2014) and nails (Karpas et al., 2005) is appropriate for long-term monitoring.
National data on concentrations of environmental chemicals, including uranium, were collected as part of the Canadian Health Measures Survey (CHMS), an ongoing national direct health measures survey launched in 2007 by Statistics Canada, in partnership with Health Canada and the Public Health Agency of Canada (Health Canada 2010, 2013 and 2015c). Uranium data for cycle 1 of the CHMS were collected between March 2007 and February 2009 from approximately 5,600 Canadians aged 6 to 79 years at 15 sites across Canada while uranium data for cycle 2 were collected between August 2009 and November 2011 from approximately 6,400 Canadians aged 3 to 79 years at18 sites across Canada. Geometric means could not be calculated for either uranium concentration levels in whole blood or in urine for both Cycle 1 and Cycle 2 since sample results were below the limits of detection (LOD for whole blood: Cycle 1 - 0.005 µg/L, Cycle 2- 0.007 µg/L, LOD for urine Cycles 1 and 2 – 0.01 µg/L) (Health Canada, 2010, 2013). Uranium was not included in Cycle 3's 2012 – 2013 monitoring program (Health Canada, 2015c).
6.0 Analytical methods
The United States Environmental Protection Agency (U.S. EPA) has approved several methods for measuring total uranium in drinking water, including both mass based and radiological (activity) methods. Mass based methods are exclusively recommended in this document, as the health effects are based on the chemical properties of uranium and calculated on a mass exposure basis. The radiological methods approved by the U.S. EPA are not appropriate for use as they rely on conversion factors to estimate the mass of uranium based on radioactivity which could lead to inaccuracies. The following approved mass based analytical methods for uranium have detection limits that vary between 0.001 and 5 µg/L:
- EPA 200.8 Rev 5.4 uses an inductively coupled mass spectrometry (ICP-MS) method and has a method detection limit (MDL) of 0.1 µg/L when the instrument is in scanning mode or 0.01 µg/L when the instrument is in selection ion monitoring mode (U.S. EPA, 1994).
- EPA 908.1 uses a fluorometric method and is suitable for determining soluble concentrations greater than 0.1 µg/L (U.S. EPA, 1980).
- SM 3125 uses an ICP-MS method and has an instrument detection limit of 0.032 µg/L for U-235 or 0.001 µg/L for U-238 (APHA et al., 1998, 2005).
- SM 7500 U-C uses a fluorometric method. Samples containing more than 20 µg/L can be determined directly, and samples with less than 20 µg/L can be pre-treated (APHA et al., 1989).
- USGS R-1180-76 uses a direct fluorometric method, with minimum detection level of 0.3 µg/L (Thatcher et al., 1977a).
- USGS R-1181-76 uses a fluorometric method with extraction procedure, with a minimum detection level of 0.01 µg/L (Thatcher et al., 1977b).
- ASTM D2907-91 and ASTM D2907-97 (withdrawn by ASTM in 2003, no replacement) use a direct fluorometric method, with a method range of 0.005 – 50 mg/L (ASTM, 1991a, 1997a).
- ASTM D5174-91, ASTM D5174-97, ASTM D5174-02 and ASTM D5174-07 use a pulsed laser phosphorimetry, with a method detection limit of 0.1 µg/L (ASTM, 1991b, 1997b, 2002, 2007).
- ASTM D5673-10, ASTM D5673-05, and ASTM D5673-03 use an ICP-MS method, with an estimated instrument detection limit of 0.02 µg/L (ASTM, 2003, 2005, 2010).
- USDOE U-04-RC is a preparation method for fluorometry (U.S. DOE, 1997a). U-04-RC prescribes method U-01-E for the determination of uranium, and it has a method range of 1-10 000 µg/L (U.S. DOE, 1997b).
Although not currently approved for regulatory drinking water monitoring by the U.S. EPA, additional analytical methods are available to reliably measure concentrations of uranium in drinking water below the MAC. These methods include an updated version of ASTM D5673-16 with and an estimated instrument detection limit of 0.02 µg/L (ASTM, 2016) and SM 3125 with an instrument detection limit of 0.032 µg/L for U-235 or 0.001 µg/L for U-238 (APHA et al., 2017). In selecting an analytical method, consideration should be given to the water matrix to minimize the effect of interfering agents. The use of the prescribed sample pre-treatment or mitigation steps can help eliminate or decrease the effects of interfering agents. It is important that analyses are undertaken by an accredited laboratory to ensure accurate results and appropriate quality assurance and quality control.
Inductively coupled plasma mass spectrometry (ICP-MS) is a highly sensitive detection technique. In ICP-MS an inductively coupled plasma source is used to ionize and atomize the analyte, which is then separated by a mass spectrometer based on mass-to-charge (m/z) ratio. The number of ions striking the detector can be translated from an electrical signal and related to the number of atoms of the analyte (USGS, 2013). ICP-MS can be subject to a number of sources of interferences from isobaric elemental interferences (where ions with the same nominal m/z ratio cannot be distinguished from the analyte of interest), and isobaric polyatomic ion interferences (where ions with more than one atom have the same m/z ratio as the analyte of interest), although none are specifically noted for the measurement of uranium (U.S. EPA, 1994). Generally, the presence of high dissolved solids in a sample may interfere with ion transmission, and interferences can occur when dissolved solids exceed 0.2% (w/v) (U.S. EPA, 1994) and 0.5% (w/v) (APHA, 1998, 2005). Elsewhere, in the literature, it has also been noted that samples with salts exceeding 0.1% should be diluted for ICP-MS (Pawlak and Rabiega, 2002).
For analysis using fluorometry, samples are fused with sodium fluoride (U.S. EPA, 1980; U.S. DOE, 1997), or a mixture of sodium fluoride, sodium carbonate, potassium carbonate (Thatcher et al., 1977a, 1977b) which are then excited with ultraviolet light and measured with a fluorimeter. Fluorometric methods are subject to inference from cations and/or anions through either quenching or enhancing the fluorescence (U.S. EPA, 1980; APHA et al., 1989; ASTM, 1997a). Quenching is also noted to occur in methods 1180-76 and 1181-76 as a result of transition metals (e.g., chromium, copper, and manganese) (Thatcher et al., 1977a, 1977b). When uranium is concentrated by precipitation with aluminum phosphate, as in SM 7500-U C (APHA et al., 1989) or U.S. EPA 908.1, carbonate ions can prevent the co-precipitation of uranium with aluminum phosphate (U.S. EPA, 1980). It is important to note that the U.S. EPA approved SM 7500 U-C method is a fluorometric method and is only found in the 17th edition of Standard Methods (APHA et al., 1989). In the 23rd edition of Standard Methods, SM 7500 U-C is an isotopic method that is meant to be used for the measurement of radiological activity.
Phosphorimetry relies on the long-lived luminescent quality of the uranyl ion, which can be excited by ultraviolet or visible light. In pulsed laser phosphorimetry, a pulsed laser acts as the excitation source and the luminescence intensity is measured (Brina and Miller, 1992). Interference can come from a variety of sources including compounds that absorb ultraviolet excitation light (e.g., ferric ion, oxy-anions) or visible excitation light (e.g., chromate), luminescence from humic acids or organic degradation products, or quenching from reducing agents such as silver, lead, iron (II), manganese (I) and thallium. In addition, samples are initially wet-ashed to remove organic impurities prior to measurement (ASTM, 2007).
The current practical quantitation limit (PQL) established by the U.S. EPA is 5 pCi/L (or approximately 3.33 µg/L – 7.46 µg/L) (U.S. EPA, 1991). In the second-six year review of existing National Primary Drinking Water Regulations, the U.S. EPA indicated that some performance evaluation and proficiency testing data were available at or below the current PQL. However, the U.S. EPA made no conclusions or changes regarding the PQL based on these data (U.S. EPA, 2009).
7.0 Treatment technology
Management strategies for uranium at the municipal-scale may include source water treatment or practices such as switching to a new source, blending and interconnecting with and/or purchasing water from another water system. The best available technologies (BATs) for the removal of uranium in drinking water are coagulation/filtration, lime softening, ion exchange (IX) and reverse osmosis (RO). In addition, activated alumina (AA) may be effective in small systems. At the residential scale, there are currently no devices certified for removing uranium, however IX and RO are expected to be effective.
7.1 Uranium occurrence as a function of water quality and chemistry
The occurrence and distribution of uranium and/or radionuclides in groundwater is controlled by geology (i.e., uranium content of the aquifer rocks) and geochemistry. The rates of leaching and dissolution processes depend on pH, partial pressure of carbon dioxide, the presence of dissolved oxygen, redox potential and the presence of complexing agents (Hess et al., 1985). In general, elevated levels of uranium will occur more frequently in waters under oxic or mixed redox and high pH conditions. U.S. Geological Survey data (Ayotte et al., 2011), indicated that uranium was also found in groundwater samples under anoxic and low pH conditions. It is also important to note that the naturally occurring, long-lived uranium-238 isotope (99.3 wt %) is a parent of radionuclide progenies such as radium. Each progeny has unique chemical chemistry, solubility, mobilization and half-life properties different than the parent's properties. Focazio et al. (2001) indicated that uranium tends to be the least mobile in oxygen-poor groundwater and strongly adsorbed to humic substances; while radium tends to be the most mobile in oxygen-poor groundwater. Therefore, occurrence of parent and daughter radionuclides in groundwater may not be found together in similar concentrations (Gilkeson et al., 1983; Zapecza and Szabo, 1986).
Uranium mobility in the environment is partially controlled by its oxidative states. Although uranium occurs in several oxidation states, the most important in nature are U(IV) and U(VI). In the U(IV) oxidation state, uranium occurs as uranous U4+ ion; and in the U(VI) oxidation state – as a uranyl (UO22+) ion. The chemical speciation of uranium in the water supply is important for the treatment processes and successful operation.
The U(IV) oxidation state is the major oxidation state in the most common uranium ore minerals: uraninite (UO2) and coffinite (USiO4). U(IV) species exist as relatively insoluble and immobile precipitates under anoxic or reducing conditions. Due to the extreme insolubility of uraninite, uranous hydroxyl complexes are usually below 0.05 µg/L and not detectable in natural waters (Langmuir, 1978). However, the U(OH)5- complex may exceed this concentration under reducing conditions and at a pH greater than 8.0. The uranous fluoride complexes may increase uraninite solubility at a pH below 4.0 and under reducing conditions (Langmuir, 1978).
Thermodynamic data (Langmuir, 1978) indicate that the UO2+ ion forms weak complexes which may be found in waters with at intermediate oxidation potential and pH levels below 7.0. UO2+ ion forms less stable complexes than the complexes formed by the U4+ and UO22+ ions with the same ligands (Langmuir, 1978; 1997).
In oxidized waters and pH levels below 5.0, uranium reacts with oxygen to form the UO22+ ion, which has a high tendency to form complexes with other ions. At a typical groundwater uranium concentration of 10-8 M, uranium in U(VI) oxidation state forms stepwise aqueous monomeric hydroxyl complexes such as UO2OH+; UO2(OH)20 (aq) and UO2(OH)3-, which dominate at pH greater than 4.0. However, at a higher uranium concentration (greater than 10-7 M), polynuclear hydroxyl complexes such as (UO2)2(OH)22+ and (UO2)3(OH)5+ may form (Langmuir, 1997). In most natural waters, the uranyl ion reacts easily with anionic ligands such as sulphate, phosphate and carbonate to form complexes. Carbonate and phosphate are important ligands that influence U(VI) adsorption and transport in groundwater. At a commonly seen groundwater sulphate concentration of 100 mg/L and phosphate concentration of 0.1 mg/L, UO2SO40 and sparingly soluble UO2(HPO4)22- complexes may be formed at a pH up to 7.0 and in the pH range 4.0-7.5, respectively. Silica may form a weak complex of UO2SiO(OH)3+ at approximately of pH 6.0 (Langmuir, 1978).
In most natural waters, uranyl ion forms strong carbonate complexes, which replace the U(VI) hydroxyl complexes above pH 6.0 and at atmospheric CO2 pressure of 10-3.5 bar (Langmuir, 1978). Carbonate complexes are significant uranyl compounds and play a significant role in the removal of uranium from contaminated water. These complexes increase the solubility and mobility of uranium in the water (Jurgens et al., 2010). For the common groundwater carbon dioxide (CO2) pressure of 10-2 atm., temperature of 25°C and at mildly acidic pH levels (5.0 to 6.5), uranium carbonate (UO2CO30 (aq)) is the predominant species. Under neutral and slightly alkaline conditions (pH range of 6.5 to7.5), the principal species is the UO2(CO3)22- complex and at pH greater than 7.5, the principle species is the UO2(CO3)34- complex. Uranyl hydroxyl complexes such as (UO2)3(OH)5+ can also be found, but generally in small percentages unless the temperature is high or if the water were depleted of carbonate and at alkaline pH (greater than 10.0) (Langmuir, 1997; Clifford, 1999).
Recent studies analyzed the chemical composition of groundwater samples and modeled the uranium speciation (Bernhard et al., 2001; Dong and Brook, 2006; Fox et al., 2006; Gustafsson et al., 2009; Prat et al., 2009; Norrstrom and Lov, 2014). Norrstrom and Lov (2014) reported the presence of two calcium-uranyl-carbonate complexes (Ca2UO2CO30 (aq) and CaUO2(CO3)32- ) in private drilled wells and found that their formation was dependent on the calcium (Ca) concentration in the water. Both complexes comprised 20-80% of the total uranium complexes in water with a pH range from 7.2 to 7.4. Other complexes found in the same pH range were neutrally charged complexes UO2CO30 (aq) (up to 19%) and UO2(OH)20 (aq) (up to 14%). A total dominance (80-100%) of both calcium-uranyl-carbonate complexes was observed at a pH greater than 7.4. The neutrally Ca2UO2CO30 (aq) comprised 45-85%; while the negative charged CaUO2(CO3)32- made up 20-50% of the total complexes under these conditions. In general, the uncharged uranium complexes constituted 50-80% of the total complexes the pH range from 6.7 to 7.8 (Norrstrom and Lol, 2014). Prat et al., (2009) also reported that the uranium speciation was mainly Ca2UO2CO30 (aq) and CaUO2(CO3)32- complexes in a pH range from 7.9 to 9.0 in drilled wells in Southern Finland. Other studies determined the formation constants for other alkali earth metals and suggested that M2UO2(CO3)3 and MUO2(CO3)32- complexes (M=Mg2+, Sr2+ and Ba2+) could have a potentially important role in the speciation and environmental behavior of uranium (Kalmykov and Choppin, 2000; Dong and Brooks, 2006).
Uranium is also readily adsorbed to dissolved organic carbon (DOC). Artinger et al. (2002) suggested that uranyl is almost completely sorbed to DOC at pH below 6.0. A fraction of uranyl-DOM complexes (0% - 60%) was observed at a pH range from 6.3 to 7.2 in groundwater samples. The large variation in the uranium organic complex fraction was attributed to the competition from other complex-forming ions with the large impact of the carbonate species. Other uranium complexes observed in the same pH range were UO2CO30 (aq) (10-36%), UO2OH+ (10-29%) and UO2H3SiO4- (4-11%) and to lesser extent UO2(CO3)34-(aq), UO2(SO4)0(aq) and UO2HPO40(aq) (Norrstrom and Lov, 2014).
7.2 Municipal scale
The U.S. EPA (2010) lists four main technologies as best available technologies (BATs) for the removal of uranium in drinking water: coagulation/filtration, lime softening, ion exchange (IX) and reverse osmosis (RO). In addition to these technologies, activated alumina (AA), point-of-use (POU)-RO and POU-IX, are identified by the U.S. EPA (2010) as Small System Compliance Technologies (SSCTs).
In order to select the appropriate treatment system for uranium removal or to optimize an existing system, it is important for utilities to have a good understanding of the uranium species present in the source water. Studies indicate that uranium complexes and their charges (negative, neutral or positive) affect the efficiency of treatment techniques such as coagulation and adsorption (Ankomah, 1991; Bang and Meng, 2004; Fox et al., 2006; Favre-Reguillon et al., 2008; Norrstrom and Lov, 2014). Norrstrom and Lov (2014) noted that to be able to identify the effective treatment techniques for uranium removal, it is important to measure the most crucial parameters responsible for uranium complex formation. Water quality parameters such as pH, alkalinity, calcium, and total organic carbon (TOC) concentrations may help to identify which uranium species dominate in the water. For the most accurate results, pH should be measured in the field at the same time that the sample is collected (Bachman, 1984).
A utility should determine if the existing treatment technology is capable of removing uranium even though it was not originally designed for this purpose. Because disposal options may be limited, systems need to be aware of the types of residuals (liquid and/or solid) that will be generated by each treatment process in order to determine whether the treatment will be practical and affordable.
In selecting a treatment technology, utilities should also consider the characteristics of the waste residual, including uranium and co-contaminant concentrations, in order to assess disposal options and regulatory requirements.
7.2.1 Control options
Control options for reducing excess uranium level in drinking water do not typically produce wastes that may cause disposal problems, do not require any additional operator training and are less expensive (U.S. EPA, 2016). Typical control options include switching to a new source, blending, and interconnecting with and/or purchasing water from another water system (U.S. EPA, 2012). Switching to another source may involve drilling a new well in an aquifer containing low uranium levels, sealing off water producing zones containing high uranium levels, or finding an uncontaminated surface water source. Before switching, attention must be given to water quality of the new source so that it does not interfere with the existing treatment process(es). Significant changes in water quality may require new treatment processes, impact the distribution system, and cause other compliance issues. Switching to another source also may be limited by the availability of new sources, existing water rights, and/or costs for transporting the new source water to the treatment plant.
Blending involves diluting uranium concentrations of a contaminated source with another source containing low concentration or no uranium. To minimize piping required to carry the sources to a common mixing point, it would be ideal for the sources to be close to each other. Blending usually occurs in a storage tank or a common header with resulting uranium concentrations below the MAC. Similar to switching to another source, care should be taken to any changes in water quality. Characterization of water quality must be carried out to ensure that changes in water quality resulting from blending are assessed and that potential impacts to the existing treatment processes and distribution system are determined (U.S. EPA, 2012). Also any change in water quality should not cause other compliance issues.
When interconnecting with another water system, the recipient system must consider a number of factors including if there is a nearby water supply that meets the uranium MAC; , if this other system is willing to interconnect or consolidate and; if the interconnecting system can handle increased demand (and associated residuals management) resulting from additional customers. The water quality from the interconnecting system should be evaluated to ensure that the water characteristics will not impact the distribution system. If the purchased (interconnected system) water is more corrosive, it may cause leaching of lead or copper in the distribution system. The impact of interconnection on water quality and the distribution system and costs are considerations in the decision-making process (U.S. EPA, 2016).
7.2.2 Conventional coagulation/filtration
Conventional coagulation/filtration is one of the most common water treatment processes used by larger water systems for removing particulates and turbidity from water supplies. The removal of uranium by a coagulation process most likely occurs through adsorption and co-precipitation of the dissolved uranyl complexes by the coagulant precipitates. The literature indicates that uranium removal efficiency is affected mainly by water pH and the coagulant dose. The optimum coagulant dose is dependent on the type of coagulant and the concentration of uranium in the raw water (Lee et al., 1982; Hansen et al., 1987; Grafvert et al., 2002; Baeza et al., 2006). In general, the uranium removal rates exceed 85% when using either alum or ferric sulphate at a pH of 6.0 and 10.0. Ferrous sulphate was not as effective at pH 6.0 (44%) but had a greater than 85% removal efficiency at pH 10.0 (Lee et al., 1982; Sorg, 1988; CH2M Hill, 2006).
Lee et al. (1982) attributed the pH dependency of uranium removal to the stability and charge characteristics of the uranyl species and the iron/aluminum hydroxide precipitates. At pH 6.0, the charge of the predominant uranyl complex (UO2CO30) and aluminum hydroxide solids are neutral, thus allowing them to agglomerate. In contrast, at pH 4.0, the charges of the predominant uranyl species (UO22+ and UO2OH+) and the aluminum hydroxide solids are positive. At pH 8.0, the charges of the predominant uranyl species (UO2[CO3]22– and UO2[CO3]34–) and the aluminum hydroxide solids are negative. Electrostatic repulsion keeps the respective uranyl complexes from being attached to the metal hydroxide solids, causing low uranium removals at pH 4.0 and 8.0. However, the high removals at pH 10.0 were attributed to the formation and attachment of the uranium complex (UO2)3(OH)5+ to the negatively charged metal hydroxide solids. Since calcium carbonate (CaCO3) precipitates at pH> 9.5, the depletion of the carbonate from the water may destabilize the uranyl-carbonate complexes and force them to hydrolyze as a positively charged complex (Lee et al., 1982; Lee and Bondietti, 1983).
Full-scale uranium removal data are limited. Uranium concentrations in the raw and treated water were analyzed at 20 coagulation/filtration plants that used either surface (17) or groundwater (3) as drinking water sources (White and Bondietti, 1983). Among these plants, one (Moffat Treatment Plant in Denver, Colorado) had a feed water uranium concentration of 15.8 μg/L, achieved a concentration of 4.0 μg/L (75% removal) using alum coagulant at pH 7.5. However, the remaining 19 plants had less than 10.0 μg/L of uranium in their source waters and little or no uranium removal was seen with iron or aluminum coagulants. Despite the low levels of uranium removal achieved by these water treatment plants, Lee et al. (1982) indicated that a more appropriate coagulant dose, an adjustment of the water pH or both may improve the removal of uranium from drinking water.
A pilot-scale study using a 1-gpm chemical clarification system and uranium-laden well water, with concentrations ranging from180.0 µg/L to 450.0 μg/L reported greater than 99.0% uranium removal by ferric chloride (Hanson et al., 1987). The study observed a difference in the uranium removal when the pH of the water was adjusted from 4.0 to 10.0. The best removals were achieved using a dose of 30.0 mg/L ferric chloride, with 99.0% removal at pH 10.0 (< 2 μg/L in the filtered water) and 81% removal at a pH of 5.8 being reported. Lower removals were observed at pH 4.0 (31.0%) and pH 8.2 (18.0%) at this same dose of ferric chloride. Increasing the ferric chloride dose from 30.0 to 90.0 mg/L only slightly increased the removal from 81.0 to 87.0% at pH 6.0 but did not improve the removal at pH 10.0 (Hansen et al., 1987; Sorg, 1988).
Based on jar tests performed on pond water containing 83 μg/L uranium, removal efficiencies in the ranges of 63 to 89% and 83 to 87% were observed at pH 6.0 and 10.0, respectively, with ferric sulphate doses ranging from 10 to 25 mg/L (Lee et al., 1982; Lee and Bondietti, 1983). Removal increased with increasing dose of ferric sulphate. However, at pH 4 and pH 8, the maximum removals observed were 18 and 43%, respectively, with a dose of 25 mg/L of ferric sulphate. Similar results were reported with ferrous sulphate coagulant in terms of uranium removal being dependent on the pH of the water and the coagulant dose applied. The range of removal efficiency achieved was 57 to 93% using a dose range of 10 to 25 mg/L ferrous sulphate at pH 10.0. However, lower removals were observed at pH 8.0 (20%), pH 6.0 (44%) and pH 4.0 (33%). With an alum dose of 10 mg/L, the removal efficiency was found to be 95% at pH 10.0; at pH 6.0 the removal rate increased with increasing alum dose with an 88% removal being observed with an alum dose of 25 mg/L. However, lower removal rates of 48% and 21% were observed at pH 8.0 and pH 4.0, respectively, using this same dose. Although the study obtained the best results for uranium removal at pH 10.0, the stability of the flocs should be considered. In practice, alum is not used at pH values below 4 or greater 10 due to increased solubility of the aluminum hydroxide solids (Lee et al., 1982).
Several limitations apply to the conventional coagulation process. The efficacy of uranium removal by coagulation/filtration process depends on water quality parameters, especially pH. While the efficiency of uranium removal increases at a higher pH, that of turbidity removal does not under these conditions. For a hard groundwater system that uses coagulant in addition to lime softening, it may be preferable to lower the water pH to 6.0 to address concerns over scaling caused by calcium and magnesium precipitating at pH 10.0. In this situation, the pH of the water would subsequently need to be adjusted (increased) prior to entering the distribution system.
The waste products from a coagulation/filtration facility include both liquid and solid wastes. The liquid waste stream is consists of sedimentation waste and backwash water. The solid waste comprises sludge (with radionuclide accumulation) and aged (spent) filtration media. The filter media usually lasts several years before replacement is required and may contain elevated levels of uranium. The uranium concentration of the media should be assessed to determine if special precautions are necessary for waste disposal (CH2M Hill, 2005). Residuals generated by conventional coagulation/filtration treatment should be assessed to determine if they need to be disposed of in accordance with the Canadian Guidelines for the Management of Naturally Occurring Radioactive Materials (NORM) (Health Canada, 2014). Clifford (2016a) has estimated that for 50% removal of 40 µg/L uranium from the influent water, the resulting dry sludge would contain 800 pCi/g Fe(OH)3(S).
7.2.3. Lime softening
Lime softening for calcium removal is typically accomplished at a pH range of 9.0–9.5, while for magnesium removal, lime and soda ash and/or magnesium carbonate are added beyond the point of calcium carbonate precipitation to form magnesium hydroxides precipitates (pH greater than 10.5). Jar tests achieved 85–90% uranium removal in drinking water by lime softening (Lee et al., 1982). Uranium removal of up to 99.0% was observed when magnesium hydroxide precipitates are formed, either from natural magnesium in the source water or through the addition of magnesium prior to the lime softening (Lee et al., 1982; Sorg, 1988).
Laboratory experiments (Lee et al., 1982; Lee and Bondietti, 1983) demonstrated that with the addition of between 50 and 250 mg/L of lime alone and a pH in the range of 10.6–11.5, the uranium removal from a pond water containing 83 μg/L could reach 85 to 90%. The indigenous magnesium concentration in the pond water was 13 mg/L. With the addition of lime doses greater than 100 mg/L and 120 mg/L magnesium carbonate, the uranium removals were increased to 99% at pH levels in the range of 10.6 to 11.3 for this specific water. The authors concluded that magnesium hydroxides precipitates play an essential role in uranium removal from natural water by the lime treatment. It was suggested that due to the carbonate depletion of the water at this high pH, the chemical state of the uranium species is converted to uranyl hydroxide (UO2)3(OH)5+, which would adsorb and/or co-precipitate with gelatinous magnesium precipitates. The results also indicated that, at a pH between 8.5 and 10.6, lower uranium removals (10 to 30%) would occur if only calcium carbonate or magnesium hydroxide precipitates were formed during the lime softening process (Lee et al., 1982).
The lime softening process is relatively expensive and may be impractical to use for uranium removal unless hardness reduction is a concurrent treatment goal. Lime softening technology requires careful monitoring to ensure proper operation. The treated water may require re-carbonation (to reduce pH) and the addition of corrosion-inhibiting chemicals to protect the distribution system (to counter potentially altered corrosivity of the treated water due to the removal of hardness and alkalinity) (U.S. EPA, 2012).
Treatment residuals generated by lime softening include sludge, backwash water and spent media. The large quantity of uranium-laden residuals generated by the process may impact disposal options and complicate the disposal process. Residuals generated by lime softening should be assessed to determine if they need to be disposed of in accordance with the Canadian Guidelines for the Management of Naturally Occurring Radioactive Materials (NORM) (Health Canada, 2014). Calculations indicated that for a 90% removal of 40 µg/L uranium, the typical dry sludge can contain 135 pCi/g CaCO3(s) (Clifford, 2016a).
7.2.4 Ion exchange
Ion exchange is a physicochemical process in which there is an exchange of ions in the raw water with ions within the solid phase of a resin (cation or anion resin). Exchange resins exhibit a degree of selectivity for various ions, depending on the concentration of ions in solution and the type of resin selected. The ion exchange capacity and the selectivity of the resin are important considerations when selecting a resin.
Both cation and anion resins have been evaluated and used for uranium removal. For uranium removal, anion exchange resins would typically exchange chloride ions for negative uranyl-carbonate complexes (UO2[CO3]22- and UO2[CO3]34-). Uranium anions are the ions most preferred by SBA resins but their effectiveness may be limited when the sulphate concentration in the source water is high. SBA resins can be operated at a wide pH range. As such, the benefit of using SBA resin is the ability to operate without pH adjustment. Cation exchange resins exchange the UO22+ cation with hydrogen, sodium, or calcium ions at acidic pH (below 5.0). Since the UO22+ cation is significant only at low pH and the process would require adjustment of the pH before and after the treatment, cation exchange is not a practical water treatment process for removing uranium (Clifford and Zhang, 1995).
SBA resin in the chloride form is very effective and considered one of the most cost-effective methods for removal of uranium especially for small community water systems supplies (Sorg 1988; Clifford, 1999; Clifford et al., 2011). Factors affecting the anion exchange process include water pH, influent uranium concentration, competing ion concentration (especially sulphate), resin type, and alkalinity.
The effectiveness of the anion exchange process for uranium removal from drinking water supplies have been studied using bench- and pilot-scale apparatus and at full-scale treatment facilities (Lee et al., 1982; Lassovszky and Hathaway, 1983; Lee and Bondietti, 1983; Hanson et al., 1987; Jelinek and Correll, 1987; Jelinek and Sorg, 1988; Sorg 1988; Zhang and Clifford, 1994; Clifford and Zhang, 1995; Clifford, 1999; Gu et al., 2004; Phillips et al., 2008; Malcom Pirnie, 2009).
Eleven small SBA systems installed in homes, schools, and community water systems at several locations in Colorado and New Mexico, received different groundwater sources containing from 22 to 104 μg/L of uranium, TDS concentrations from 166 to 1,200 mg/L and sulphate concentrations from below 5 to 408 mg/L. After two years of continuous (360 gpd; 1.36 m3/d) or intermittent (37 gpd; 0.14 m3/d) system operation, seven systems produced from 7,900 to 62,900 bed volumes (BVs) treated water with uranium concentrations below 1.0 µg/L and reached uranium removals from 98.6 to >99.9%. The highest BVs were achieved by two of the continuous systems: 62,000 BVs (99.6% removal) and 21,200 BVs (99.9% removal) after treating influent uranium concentrations of 28 µg/L (3.0 mg/L sulphate) and 64 µg/L (124.0 mg/L sulphate), respectively. These studies demonstrated that the SBA resins had high selectivity and capacity for uranium and treated various raw water qualities for long periods of time (Fox and Sorg, 1987; Sorg, 1988). A small-scale anion exchange system consisting of two parallel cartridge pre-filters and two pressure vessels filled with a SBA resin in series, was installed at elementary school in Jefferson County, Colorado. The system was capable of treating approximately 285,000 gallons of raw water with an influent uranium concentration in the range from 40 to110 µg/L. The first vessel continuously produced treated water with uranium concentrations below 1 µg/L during the six-month study period (Jelinek and Sorg, 1988). Another small-scale system effectively reduced uranium concentration of up to 200 µg/L in groundwater to 2 µg/L with a run length of 85,000 BVs (Tamburini and Habenicht, 1992). Pilot-scale studies, performed in New Mexico, using selected anion exchange resins consistently removed 99.0% of the influent uranium levels (measured as 86 pCi/L–120 pCi gross alpha/L). A treatment goal concentration of 1.0 pCi/L uranium (0.7–1.5 µg/L) was not observed at 64,000 BVs with a loading rate of 5 gpm/ft3 (11.1 L/m/s) and at 33, 000 BVs with a loading rate of 2.5 gpm/ft3 (5.6 L/m/s). The results from another pilot-scale study conducted in Arizona were consistent with above results. An influent uranium activity of 13 pCi/L (8.7–19.4 µg/L) to 38 pCi/L (25.3–56.7 µg/L) was reduced to below 1pCi/L (0.7–1.5 µg/L) while treating greater than 60,000 BVs at both flow rates (Jelinek and Correll, 1987).
Work performed by Zhang and Clifford (1994) confirmed that SBA resins in the chloride-form possessed exceptionally high capacities for UO2(CO3)22- and UO2(CO3)34- complexes. A macroporous polystyearene quaternary-amine SBA resin exhibited high capacity for removing uranium from groundwater with a total hardness of 150.0 mg/L as CaCO3, chloride concentration of 47 mg/L and a sulphate concentration below 1.0 mg/L at temperatures ranging from 20–24°C. A pilot-scale column with an EBCT of 3.0 minutes received feed water with 110.0–125.0 μg/L of uranium concentrations at pH 7.6 to 8.2. The ion exchange unit treated water to uranium levels below 6.0 µg/L with run length of 302,000 BVs (478 days). Uranium concentrations below 1.0 µg/L were observed with run lengths of 260,000 BVs (99.2% removal) and beyond this run length, the uranium leakage in the treated water slowly increased at a rate of 1.0 µg/L per 10,000 BVs. The total uranium loading on the column was 30 g/L resin, corresponding to 7.8 ×104 pCi/g dry resin (equivalent to 2.21 lb U3O8/ft3 anion resin). Generally, such long runs would not be used in water treatment practice due to the fouling and waste disposal problems (Zhang and Clifford, 1994).
Uranium and radium are often found in close proximity due to the same natural radioactive decay chain. Scott and Barker, (1969) revealed numerous cases of the simultaneous presence of uranium and radium in the water supplies. In a pilot-scale study, a combined ion exchange process was evaluated for a simultaneously removal of radium and uranium from groundwater (Clifford and Zhang, 1995). The process used was a modified ion exchange softening, in which an appropriate amount of SBA resin was added to a water softener to reduce 25.0 pCi/L radium and 120.0 µg/L uranium in groundwater. The tests were conducted with mixed (mix of strong acid cation resin [SAC] and SBA resin) and stratified (layer of lighter anion resin over cation resin) beds. The beds were tested either in single-use and cyclic modes. A virgin mixed bed (25% SBA/75% SAC), was run to exhaustion with radium breakthrough (5 pCi/L) occurring at about 2,100 BVs, which correspond to 2,830 BVs for the SAC bed alone; while the uranium breakthrough (20 µg/L) was not reached until 65,000 BVs, corresponding to 260,000BVs based on SBA resin. The mixed bed operated for 309 days with a total BVs throughput of 135,000 BVs, corresponding to 540,000BVs for the SBA resin and total uranium loading of 40 g/L anion resin. Tests conducted with different mixed depth beds (2.5, 5.0 and 10% of SBA resin) showed that 5-10% anion resin in a mixed bed would be adequate for combined uranium and radium removal by water softening treatment. In general, the mixed bed produced less uranium and radium leakage in the treated water than the stratified bed when both beds were tested in cyclic down- flow regeneration mode (Clifford and Zhang, 1995).
A predictive computer model showed that a decrease of the influent uranium concentration from 120 µg/L to 20 µg/L would increase the run lengths from 300,000 BVs to 815,000 BVs. This high sensitivity of the run lengths to the uranium concentration was explained by the fact that uranium is a highly preferred species and occupies a significant fraction of the resin sites at exhaustion (Zhang and Clifford, 1994). Significant water pH changes can greatly affect uranium removal due to the changes of the uranium carbonate complexes and the available carbonate concentration in the feed water. Zhang and Clifford, (1994) reported that uranium removal was not impaired when the pH was lowered from a range of 7.6–8.2 to 5.8. The researchers attributed the unexpectedly good performance at this lower pH to conversion within the resin of UO2CO30 (dominant species at 5.8) to UO2(CO3)22– and UO2(CO3)34– using the HCO3– ions present in the feed water. However, when the pH was further reduced to 4.3, uranium leakage occurred immediately after system startup and a virgin column was completely exhausted at 6,000 BVs, presumably caused by the formation of UO22+ (Zhang and Clifford, 1994).
Traditional SBA resins are impacted by sulphate ions. The computer simulation model predicted that the run length would be significantly reduced from 300,000 BVs to 135,000 BVs if sulphate concentrations were increased from 0 to 64 mg/L (Zhang and Clifford, 1994). Another factor that would be of concern when using ion exchange process is chromatographic peaking, which causes the less preferred ion to exit the resin bed at a higher concentration than their influent concentration. Because uranyl-carbonate complexes possess high affinity to SBA resins, chromatographic peaking should not be a major concern, relative to other anionic contaminants, such as arsenic. No uranium chromatographic peaking has been reported in the literature.
Typically, when a resin bed is exhausted, it is regenerated with a concentrated solution of ions initially exchanged from the resin. In spite of the high affinity for uranyl-carbonate complexes, regeneration of ion exchange resins can be easily accomplished with saturated sodium chloride (NaCl) solution. The uranium recovery efficiency from the exhausted SBA resin is strongly dependent on the NaCl concentration. In general, increasing the NaCl concentration results in increased uranium recovery at a fixed regeneration level (i.e., the mass of the NaCl used). Further increasing the regeneration level beyond 4 eq Cl/eq resin (423 g NaCl/L resin) would not improve the recovery. Zhang and Clifford, (1994) observed that the addition of NaOH to the NaCl regenerant solution greatly reduced recovery of the uranium. This was attributed to the formation of uranium precipitates at high pH levels. The precipitates that were formed (i.e., sodium pyearouranate and uranium oxides) were retained in the resin pores or stuck to the resin structure and not eluted by the regenerant. A reduction in regeneration efficiency was also observed when Na2CO3 and NaHCO3 were added to NaCl solution (Zhang and Clifford, 1994).
Treatment residuals generated by ion exchange may include brine, backwash water, rinse water, and spent resins. The quantity and the concentration of uranium in the waste streams are site specific and depend on the method of plant operation and the type of regeneration used (U.S. EPA, 1996). Most conventional SBA resins have a high uranium removal capacity and treatment runs can last as long as year or more. Utilities may decide to regenerate the resin bed more frequently to produce regenerants containing lower levels of uranium in order to meet applicable disposal requirements. Clifford (1999) indicated that a cyclic run length ranging from 30,000 to 50,000 BVs would be appropriate for uranium removal in drinking water. Calculation indicated that if the feed water contains 40 µg/L the waste brine will contain approximately 80,000 pCi/L for a 30,000 BVs run length (Clifford, D.A., 2016a). However, frequent regeneration of the exhausted SBA resin can result in more chemical consumption and more liquid waste (such as spent regenerant and rinse water) and, perhaps, a shorter resin life (due to attrition and/or loss).
Since long runs with SBA resin may produce a liquid waste stream containing high levels of uranium, utilities may choose to regenerate more frequently than needed for uranium removal (i.e., after only a few hundred BVs). However, this has been shown to cause corrosion issues (i.e., leaching of copper and lead) (Lowry, 2009, 2010) because ion exchange reduces alkalinity and causes the treated water pH to be lowered during short runs (Clifford, 1999; Wang et al., 2010b).
To avoid waste streams containing high levels of uranium, which are not suitable for disposal to a sanitary sewer system, utilities may prefer not to regenerate the exhausted resin. The ion exchange process using a single-use resin is less expensive, easier to operate and maintain than a process that includes regenerating the resin. Single-use SBA resin does not require equipment and chemicals necessary for resin regeneration and it does not generate any liquid waste. The only waste product from this process is the exhausted media. These considerations make single-use ion exchange process a more viable option for uranium removal.
A few limitations exist when using anion exchange technology. Freshly regenerated IX resin removes bicarbonate ions, causing reduction in pH and total alkalinity during the initial 100 BVs of a run. The process will typically reduce alkalinity and lower the treated water pH to less than 6.5 during the first 100 BVs and the treated water pH will reach the same level as the pH of the feed water after approximately 400 BVs. Raising the pH of the treated water may be required at the beginning of the run (100–400 BVs) to avoid corrosion and resulting lead and copper problems in the distribution system (Clifford, 1999; Wang et al., 2010b; Clifford et al., 2011). Anion exchange resins can be damaged by oxidants such as chlorine. If pre-chlorination cannot be avoided, the chlorine dose rate must be carefully controlled and monitored so that the chlorine concentration entering the ion exchange system is low. Resin fouling also may be a concern if the source water contains elevated levels of particulates, metals (such as iron and manganese), and/or dissolved organic matter (DOM). To preserve the bed life, pretreatment may be needed to remove these organic and inorganic foulants. The use of pretreatment will add complexity to the system, increase costs, and, perhaps, complicate residual disposal.
7.2.5 Membrane filtration
Reverse osmosis is a process that moves treated water (or permeate) across a semi-permeable membrane against the concentration gradient, when a pressure higher than the osmotic pressure is exerted on the side with the concentrated solution. The remainder of the feed water along with rejected contaminants is discharged as a concentrated waste stream. The effectiveness of the process and the amount of reject water produced are heavily dependent on the water quality, membrane properties, and size and charge of contaminant ions. Because the presence of chlorine residuals, particulates, and scale-forming ions (i.e., calcium, barium, iron, and silica), in the feed water can adversely affect RO performance, pretreatment is often required to preserve membrane life.
Information on the effectiveness of RO technology to remove uranium in drinking water is limited at the municipal scale. However, studies involving pilot- and full-scale evaluations of the effectiveness of commercially available POU-RO units for uranium removal at the residential-scale are discussed in Section 7.5.
A pilot-scale study (Huxstep and Sorg, 1988) evaluated the performance of four RO membranes for uranium removal in groundwater with average concentrations in the range 277 to 682 μg/L. The membranes operated under different operating conditions (pressure and water recovery) according to the manufacturers' specifications. All four membranes achieved greater than 99.0% removals of uranium for up to 74 days of intermittent system operation. Uranium concentrations were reduced to 2.8–6.8 μg/L, on average.
Nanofiltration (NF) membranes have been tested for their efficiency to remove uranium from drinking water. Studies indicated that the NF membranes effectively rejected (95–98%) uranyl-carbonate complexes UO2(CO3)22– and UO2(CO3)34– which most commonly occur in natural waters (Raff and Wilken, 1999; Favre-Reguillon et al., 2008; Malcolm Pirnie, 2009). A pilot-scale study evaluated two commercially available flat sheet NF membranes to remove uranium and gross alpha activity in groundwater. The membranes reduced a feed uranium concentration of 44 µg/L to a range of 1.0 to 2.0 µg/L (95% to > 98% rejection) when they were operated at both 30% and 80 % recovery. As expected ion rejection was higher when the membranes operated at 30% recovery (Malcolm Pirnie, 2009). Laboratory tests conducted with an NF flat, thin film composite membrane (MWCO 150–300 Da) achieved 95% rejection of uranium from a spiked feed concentration of 20.0 µg/L with a permeate (i.e., treated water) uranium concentration below 1.0 µg/L. The rejection was due to the charge effects between the uranyl-carbonate complexes and the charged surface of the membrane (both negatively charged) and dependent on the ionic strength (Favre-Reguillon et al., 2008).
Despite limited information, both RO and NF membrane processes have been shown to be effective for the removal of uranium in drinking water. However, disadvantages of RO include higher costs due to the energy needed for achieving the high pressure required and the extensive pre-treatment to preserve the membrane's life. Another disadvantage is the production of a large amount of reject water (ranging from 10% to 70%) (U.S. EPA, 2012). NF membranes can remove uranium with lower energy requirements and with higher water recovery (less reject water) as compared to RO membranes. Site-specific testing is recommended to determine the design criteria, potential fouling and pre- and post-treatment needs when utilities consider NF treatment.
Limitations of the RO process include possible membrane scaling, fouling, and failure as well as higher energy and capital costs. Calcium, barium, and silica can cause scaling and decrease membrane efficiency. Colloids and bacteria can cause fouling. Both scaling and fouling will increase pressure drop, thus decreasing membrane life and increasing energy costs. Pre-treatments such as softening and cartridge filtration and/or membrane cleaning can help obtain acceptable membrane run times. Chlorine can damage RO membranes and should be quenched using de-chlorination chemicals or GAC. Since RO continually and completely removes alkalinity in water, it will continually lower product water pH and increase its corrosivity. Therefore, the product water pH must be adjusted to avoid corrosion issues in the distribution system such as the leaching of lead and copper (Schock and Lytle, 2011; U.S. EPA, 2012).
The waste products from the membrane processes include both liquid and solid residual. The liquid residual streams are comprised of backwash wastewater from the pre-treatment membranes, brine from the RO membranes as well as waste from clean-in-place chemicals. The solid residual consists of spent membranes with a potential of radionuclides contamination (U.S. EPA, 1996; 2004). The quantity and concentrations of uranium in rejected water depend on the concentration of the contaminant in the source water, the removal rate and the fraction of the water rejected. A pilot plant RO study reported uranium concentrations ranging from 301 µg/L to 1,125 µg/L in rejected water from three different RO membranes. The broad range was a result of the wide range of uranium concentration in the feed water (154–682 µg/L) and recovery rates from 10.4% to 50% of the individual membrane tested (U.S. EPA, 1996).
7.2.6 Activated alumina and other adsorptive media technology
Although listed as SSCT, activated alumina (AA) is not very effective in removing uranium from water supplies, based on results of a bench-scale study performed by the U.S. EPA. The results indicated that the run lengths were not as high as with anion exchange resins. During a six-cycle test run, only 1,600 to 2,000 BVs were achieved before reaching initial breakthrough at around 1 μg/L (the feed water contained 273–432 μg/L of uranium) (Clifford and Zhang, 1995).
Studies investigating granular activated carbon (GAC) for uranium removal found that GAC had only limited capacity for uranium removal and that adsorption efficiency strongly depended on water pH (Lee et at., 1982; Clifford and Zhang, 1995). In a field test, four different types of GAC were tested at various water pH levels. At pH in the range 7.8–8.2, all types of GAC achieved less than 5.0% removal of uranium from a feed concentration in the range 67.0–90.0 µg/L. Improved uranium removal was observed at pH 5.8, where the predominant uncharged uranium carbonate complex UO2CO3 was readily removed by adsorption onto GAC. The effluent concentrations reached 10.0 µg/L at 800–5,000 BVs and 20.0 µg/L at 2,500–8,500 BVs depending on the type of GAC tested (Clifford and Zhang, 1995). Lee et al., (1982) reported similar results in terms of the dependency of GAC removal efficiency on the water pH. A 95.0% removal was achieved at pH below 6.4, while the lowest removal of 1% was reported for pH greater than 9.1.
In recent years, a number of single-use absorptive media (AM) products, such as iron-based media (granular ferric oxide [GFO] and granular ferric hydroxide [GFH]), hybrid ion exchange resin (HIX), titanium-based media (Metsorb G and Adsorbsia GTO) have been developed for arsenic removal and have demonstrated an ability to remove uranium in drinking water (Katsoyiannis, 2006; Westerhoff et al., 2008; Yusan and Akyil, 2008;Coonfare et al., 2010; Wang et al., 2010a; Chen et al., 2011a). ArsenXnp, a macro-porous polystyrene/divinylbenzene media, utilized hydrous iron oxide nanoparticles (for arsenic removal) impregnated into a SBA resin (for uranium removal). A full-scale ArsenXnp system was found to remove an average of 33.2 µg/L uranium from a California groundwater to below 0.1 μg/L at pH 6.9. The system consisted of two bag-filters for sediments/particles removal, followed by two parallel HIX media vessels. The single-use vessels treated 32,000 BVs with average flow rate 23 gpm (1.45 L/s) and an average EBCT of 8.8 minutes each (Wang et al., 2010a).
A longer run length was achieved during a Rapid Small-Scale Column Test which also used ArsenXnp media to reduce a uranium concentration of 56.0 µg/L in groundwater (Westerhoff et al., 2008). Uranium concentrations of greater than 1.0 µg/L were not observed even in the samples collected when complete arsenic breakthrough occurred at 50, 000 BVs. However, ArsenXnp is no longer available on the market. Similar hybrid SBA iron oxide media are available from other suppliers for the removal of arsenic from drinking water (e.g., ResinTech, LayneRT, LeWatit). However, no specific data on uranium removal was provided for these AM.
At another U.S. EPA arsenic demonstration site in New Mexico, two vessels in a parallel configuration loaded with the iron-based AM AD-33 removed an average uranium concentration of 39.3 µg/L in groundwater to below 20.0 μg/L. During the evaluation period, the average flow-rate was 114 gpm (7.2 L/s), corresponding to an EBCT of 4.7 min. To enhance arsenic removal, the feed water pH of 8.5–8.8 was adjusted to 7.0–8.0 using carbon dioxide. However, the treatment system experienced periodic losses of pH control due to the lack of a constant CO2 supply, resulting in real-time pH values cycling between 7.0 and 8.0 and over 9.0. Elevated uranium concentrations, often higher than the corresponding source water concentrations, were measured in the treated water during a number of sampling events, presumably due to the loss of pH control during system operation (Coonfare et al., 2010).
Single-use AM generally achieve lower BVs compared to conventional SBA resin (Wang et al., 2010a). This may be a consideration when assessing overall cost and operational requirements. The effectiveness of AM products is affected primarily by factors such as uranium concentration, pH, competing anions in the source water and the type of the AM product. Each AM type has unique service characteristics, such as zeta potential and point of zero charge (PZC) that are related to the base material. Measured in millivolts (mV), zeta potential is the charge that develops at the interface between a solid surface and its liquid medium. PZC is the pH value at which a solid in a liquid medium exhibits zero net electrical charge on the surface. Therefore, when the liquid medium pH is lower than the PZC, the solid surface is positively charged. Conversely, when the liquid medium pH is higher than the PZC, the solid surface is negatively charged. For example, the PZC is approximately 8.0 for iron oxides/hydroxides (Bang and Meng, 2004; Ankomah, 1991), 5.8 for titanium dioxide (Wazne et al., 2006), and 3.0 for amorphous silica (Persello, 2000). Therefore, at pH values typically encountered in water treatment plants (between 7.0 and 8.0), where uranium exists predominantly as UO2(CO3)22– and/or UO2(CO3)34–, substantial removals are expected only when using iron oxides/hydroxides.
One key limitation of the AM technology is media integrity. Some media can disintegrate and produce fines and if significant fines are produced, the media beds must be backwashed periodically to remove them. Frequent backwashing, however, can adversely affect the media due to attrition (Westerhoff et al., 2008; Coonfare et al., 2010). For some media, adjusting influent pH using an acid or a base can increase media capacity and prolong media life. However, adjusting pH adds a complexity to system's operations and maintenance. The pH of the treated water may also need to be adjusted to avoid corrosion in the distribution system. Additionally, some media may dissolve if the pH is too acidic or basic. Similarly to IX resins, some media can be regenerated, but the regeneration also adds complexity to a system's operations and maintenance, including liquid waste disposal. As a result, single-use media are generally preferred by water utilities (Wang et al., 2010a). When using a media to remove multiple contaminants, issues with optimizing removals for all contaminants can be complex. For example, arsenic removal by activated alumina is optimum at a pH between 5.5 and 6.0. At this pH, however, uranium exists as a neutral molecule and may not be removed as effectively.
7.2.7 Other technologies
Other water treatment technologies for uranium are being studied but are still primarily in the experimental stage and/or have no published information on the effectiveness of full-scale application.
7.2.7.1 Other membrane technologies
A ligand-modified, polyelectrolyte-enhanced ultrafiltration (LM-PEUF) process uses organic ligands that selectively complex the target ions and electrostatically bind them to cationic polymer, producing a filtrate with a low concentration of the target ion. Many inorganic ligands form complexes with metals in aqueous solution. Of particular interest for inorganic-ligand modified (ILM-PEUF) studies for uranium removal, are multivalent anionic U(VI) carbonate complexes such as UO2(CO3)34-. In laboratory experiments, ILM-PEUF membranes showed potential for uranium removals (e.g., >99.6% removal from 0.1 mM to below the detection limit of 0.355 μM uranium) using polyelectrolyte and carbonate (Roach and Zapien, 2009).
In the direct contact membrane distillation (DCMD) process, another type of membrane process, the saline water, containing the contaminants of interest, is passed on one side of a hydrophobic, porous membrane while a colder water stream flows on the other side to directly condense the permeate water (Yarlagadda et al., 2011). A study investigating DCMD reported greater than 99% rejection of uranium from10 to 400 µg/L from synthetic brackish water.
7.2.7.2 Titanium dioxide adsorption
A novel nanocrystalline titanium dioxide was used to treat uranium contaminated groundwater. The adsorbent was capable of reducing both uranium concentrations of 5.2 and 0.7 mg/L to below 10 µg/L achieving approximately 8,000 BVs and 11,000 BVs, respectively. The study reported a reduced capacity of titanium dioxide to remove uranium from water in the presence of inorganic carbonate at pH greater than 6.0. The authors concluded that the reduced uranium removal in the presence of carbonate was not the result of competition for adsorption site by carbonate and uranium, but rather from the aqueous complexation of U(VI) with inorganic carbonate. The nanocrystalline titanium dioxide exhibited adsorption capacity 4 times greater than the capacity of the commercially available titanium dioxide (Wazne et al., 2006).
7.2.7.3 Clinoptilolite adsorption
Several authors evaluated the feasibility of natural clinoptilolite for uranium removal from drinking water (Akyil et al., 2003; Aytas et al., 2004; Camacho et al., 2010). Camacho et al. (2010) found that the silica to alumina ratio for the specific clinoptilolite tested in their study (Si/Al = 5.0) had relatively high negative charges thus, attracting the positively charged hydroxide uranium (VI) species in water. The study showed that the natural clinoptilolite can effectively absorb uranium from water at different pH levels and different uranium concentrations. The maximum uranium removal of 95.6% was achieved at an initial uranium concentration of 5mg/L and pH of 6.0. The minimum adsorption (not reported) was observed at pH 9.0 (Camacho et al., 2010). A study reported that manganese oxide coated zeolite effectively adsorbed U(VI) from aqueous solution (Han et al., 2007). The adsorption capacity was strongly dependent on the initial pH, feed uranium concentration and the presence of competing ions such as Cu(II) and Zn(II).
7.3 Residuals management
To assess disposal options and regulatory requirements, systems need to characterize the waste stream (residuals) generated by taking into consideration the treatment technology used, the characteristics of the source water including the influent uranium concentration, the presence of co-occurring radioactive isotopes and the concentrations of other contaminants in the waste residual. Treatment technologies produce a variety of solid waste residuals (e.g., spent resin, spent filter media, spent membranes, sludge) and liquid waste residuals (e.g., brine, backwash water, rinse water, acid neutralization streams, concentrate). The characteristics and the uranium concentration in the residuals will vary with the treatment technology used and its efficiency (e.g., frequency associated with media replacement, regeneration, and filter backwash). Utilities should conduct pilot tests of the treatment technologies to determine, for example, the regeneration schedule when using IX and associated waste residuals. Despite the fact that uranium naturally emits low levels of radiation, radioactivity can vary in the residuals generated, depending on the factors listed above, and special precautions may be required when this waste stream is treated, stored, disposed of or transported. Operators may need special training to deal with these residuals.
Residuals generated by drinking water treatment facilities should be assessed to determine if they need to be disposed of in accordance with the Canadian Guidelines for the Management of Naturally Occurring Radioactive Materials (NORM) (Health Canada, 2014). The appropriate authorities should be consulted for the disposal of liquid and solid waste residuals from the treatment of drinking water containing radionuclides. A list of provincial and territorial radiation protection regulatory authorities can be found at: Federal Provincial Territorial Radiation Protection Committee (FPTRPC).
A web-based tool can also be used to estimate the removal efficiency of radionuclides and co-contaminants from drinking water and estimate the radioactive concentration in the waste residual (U.S. EPA, 2005). Residential-scale POU devices are generally not expected to contain enough radioactivity to warrant special precautions by homeowners.
7.4 Uranium in the distribution system
Accumulation of uranium in distribution system piping may occur and is influenced by a variety of factors, including uranium concentration, pipe material, co-occurrence of iron and manganese in the pipe scale deposits, pH and redox conditions in the distribution system.
Studies assessed the accumulation of several inorganic contaminants and radioactive isotopes in drinking water distribution systems (Friedman et al., 2010; Lytle et al., 2014). Lytle et al. (2014) found that total uranium levels in the solids samples (pipe scale deposits and hydrant flush solids) collected from 12 different water utilities ranged between 0.9 pCi/g and 17.0 pCi/g (estimated concentration ranged from 0.6 to 25.3 µg/g). The authors indicated that uranium was the smallest contributing element for the total sum of radioactivity (sum of gross alpha, gross beta, radium, thorium and uranium) in any individual solid sample. 233/234U isotopes were the dominant fraction of total uranium with levels ranging between 0.4 and 9.0 pCi/g (average 3.8 pCi/g [2.5–5.7 µg/g]). The 238U isotope was the second most abundant isotope with a concentration ranging between 0.3 and 5.5 pCi/g (average 1.9 pCi/g [1.3–2.8 µg/g]). The third most abundant uranium isotope was 235U with concentrations ranging between 0.0 and 3.0 pCi/g (average 0.6 pCi/g [0.4–0.9 µg/g]). In most samples the 236U isotope was measured at the detection limit (not reported). The distribution of 233/234U, 238U, 235U, and 236U to total uranium was 60%, 30%, 9%, and 1%, respectively.
Uranium can enter the drinking water distribution systems in soluble or insoluble form. Friedman et al. (2010) and Lytle et al. (2014) reported that the factors most strongly influencing uranium accumulation in the distribution system appear to be uranium concentration in the water and the co-occurrence of iron and manganese solids in scale deposits on distribution system pipe walls. Of the 12 inorganic constituents studied by Freidman et al. (2010), uranium was the eighth most concentrated trace element found in scale deposit samples, with a median concentration of 0.95 μg/g (0.0001 wt %). Uranium concentrations greater than 15.5 µg/g (approximately 0.002 wt %) were measured in scale deposit samples from pipes such as unlined cast iron, steel and galvanized pipes which were exposed to uranium concentrations of 0.4–110.0 µg/L in the treated waters and had a predominance of iron and/or manganese in the scale matrices. The highest accumulated uranium concentration of 113.0 µg/g (0.01 wt %) was measured in scale deposit formed on a high-density polyethylene (HDPE) pipe. The pipe specimen was exposed to water with an average uranium concentration of 110.0 µg/L for a period of eight years and had a thick layer of manganese-rich deposit (Friedman et al., 2010). Similarly, Friedman et al., (2016) reported a low level of uranium present in solids collected from hydrant flushing. The mass of solids collected ranged between 4.36 g and 21.6 g. The total mass of uranium measured in the solids ranged from 1.0 µg to 14.0 µg (0.00001 to 0.0001 wt%). Based on these results, the accumulation of uranium in distribution systems is not considered significant relative to other common matrix elements such as iron, manganese, calcium and aluminum.
Uranium in the U(VI) oxidation state (prevalent in chlorinated water) (Friedman et al., 2010) can precipitate, and adsorb to the solids (i.e., corrosion by-products) or sediments or can accumulate in biofilm (Dodge et al., 2002; O'Loughlin et al., 2003; Shuibo et al., 2009; Peng and Korshin, 2011; Lytle et al., 2014; Stewart et al., 2015). Iron minerals, including those associated with corroding iron from drinking water mains, exhibit a strong tendency to adsorb and accumulate uranium (Schock et al., 2005; Lytle et al., 2014). In laboratory experiments, Dodge et al. (2002) investigated the association of uranium with the amorphous and crystalline forms of iron oxide compounds commonly formed on corroded steel surfaces. The authors reported that U(VI) adsorbed and co-precipitated with the iron oxides at concentrations ranging from 4.8 µg/mg (0.5 wt%) green rust (mixed ferric/ferrous hydroxides) to 6.8 µg/mg (0.7 wt%) lepidocrocite mineral [γ-FeO(OH)] (Dodge et al., 2002). However, O'Loughlin et al. (2003) observed that a green rust might reduce U(VI) to U(IV) and immobilize uranium as enmeshed nanoparticles (UO2).
An ineffective removal of iron from raw water containing uranium could result in the passage of radionuclide-containing particles directly into the distribution system (Shock et al., 2005). Uranium has been reported to migrate in groundwater as extremely small colloids or nanoparticles of varying composition such as phosphates, iron oxyhydroxides, clays, and/or iron-coated clays (De Putter et al., 2002; Painter et al., 2002; Wieland et al., 2004). These small particles are of potential concern in drinking water since they can pass through the treatment processes, and accumulate in the distribution system (Schock et al., 2005).
Since phosphates can form chemical precipitates with a variety of oxidized metals (Friedman et al., 2010), the formation of uranyl-phosphate crystalline phases is also a potential accumulation mechanism for uranium in the distribution system (Arey et al., 1999; Dodge et al., 2002; Fuller et al., 2002). Hydroxyapatite, a common phosphate mineral deposit formed in drinking water systems, has been shown to adsorb uranyl complexes, resulting in formation of several insoluble compounds such as autunite, meta-autunite, and phosphuranylite (Fuller et al., 2002). Peng and Korshin (2011) found that uranium was associated with the mobile (31.2%), organic (22.6%) and carbonate (16.2%) fractions of corrosion scale, and that approximately 50% of the uranium was found to be in the dissolved fraction. The notable contributions of organic-bound fractions (22.6%) of uranium may indicate that biofilms and natural organic matter in the distribution system play an important role in the accumulation of uranium (Peng and Korshin, 2011). A previous study found that calcite (CaCO3) and other solid carbonate minerals strongly sorb uranium. Such minerals are common in corrosion scales and especially in the hydrant flush solids (Rihs et al., 2004).
Mobilization of uranium from the pipe scales could be initiated by several factors including water quality parameters such as pH changes (sorbed uranium), change of the oxidation conditions that could oxidize an immobilized U(IV) to a soluble U(VI) species, microbial transformation of the iron oxide or oxyhydroxide substrate (Schock et al., 2005). The presence of phosphate in the water may compete with U(VI) for surface sites on iron oxides or compete with the iron surface sites for coordination of U(VI) by forming aqueous U(VI)-phosphate complexes. These effects decrease U(VI) adsorption on iron oxyhydroxides and can cause its mobilization (Cheng et al., 2004; Schock, 2005; Friedman et al., 2010).
Friedman et al., (2010) reported an estimated uranium mass of 0.56 lb accumulated on a 100 mile pipe length (based on a 12 inch diameter pipe). The authors noted that theoretically 60% to 85% of the scale deposit would need to be released to exceed the U.S. EPA's drinking water standard for uranium of 30.0 µg/L.
7.5 Residential scale
In cases where uranium removal is desired at the household level, for example when a household obtains its drinking water from a private well, a residential drinking water treatment device may be an option for reducing uranium concentrations in drinking water.
Health Canada does not recommend specific brands of drinking water treatment devices, but it strongly recommends that consumers use devices that have been certified by an accredited certification body as meeting the appropriate NSF International (NSF)/American National Standards Institute (ANSI) drinking water treatment unit standards. These standards have been designed to safeguard drinking water by helping to ensure the material safety and performance of products that come into contact with drinking water. Certification organizations provide assurance that a product conforms to applicable standards and must be accredited by the Standards Council of Canada (SCC). In Canada, the following organizations have been accredited by the SCC to certify drinking water devices and materials as meeting NSF/ANSI standards (SCC, 2018):
- CSA Group (www.csagroup.org);
- NSF International (www.nsf.org);
- Water Quality Association (www.wqa.org);
- UL LLC (www.ul.com);
- Bureau de normalisation du Québec (www.bnq.qc.ca); and
- International Association of Plumbing & Mechanical Officials (www.iapmo.org);
An up-to-date list of accredited certification organizations can be obtained from the SCC (2018).
Although there are no certified residential treatment devices for the reduction of uranium from drinking water, there are several treatment technologies that can be effective for uranium removal at the residential-scale. Available data discussed under Sections 7.2.4 and 7.2.5 suggests that ion exchange and RO are effective and can achieve treated uranium concentrations below the MAC in the home's drinking water.
Selection of the most effective treatment system for a household will depend on a variety of factors including the concentration of uranium and other parameters such as hardness, iron, alkalinity and sulphide present in the source water.
A technology demonstration project (Lewis et al., 2007) evaluated the effectiveness of a POU-RO in removing arsenic, nitrate, and uranium at nine participating residences. The treatment process consisted of POE water softeners and POU-RO unit. Softening of the source water was performed as a pre-treatment to meet the feed water quality requirements for the RO unit. The POU-RO unit consisted of a pre-filter and an RO module with a thin film composite, semi-permeable membrane element. The POU-RO units were capable of producing up to 35.5 gpd (0.13 m3/d) of permeate water and operated at 37.0% recovery. Uranium was removed from 23.4 to 31.0 μg/L in raw water to below 0.1 μg/L in the treated water.
Huikuri et al. (1998) evaluated a small commercial POE-RO system for simultaneous removal of uranium and salinity from bedrock water supplying a private home. During an eight-month period, the spiral wound thin film composite RO membrane (100Da) removed greater than 99.0% of the uranium feed concentrations of 90.0 µg/L.
RO systems are intended for POU installation, as larger quantities of influent (incoming) water are needed to obtain the required volume of treated water, which is generally not practical for residential-scale point-of-entry systems. RO systems should only be installed at POU as the water they have treated may be corrosive to internal plumbing components. A consumer may need to pre-treat the influent water to reduce fouling and extend the service life of the membrane.
Ion exchange treatment systems for residential use are typically designed and constructed for POE installation by drinking water treatment system providers or dealer. Health Canada strongly recommends that any chemicals and components used in these treatment systems be certified to NSF/ANSI Standard 60 (NSF/ANSI, 2017a), 61 (NSF/ANSI, 2017b) and 372 – (NSF/ANSI, 2016). Reverse osmosis systems are covered in NSF/ANSI Standard 58 (NSF/ANSI, 2017c).
Before a treatment device is installed, the water should be tested to determine general water chemistry and verify the presence and concentration of uranium. Periodic testing by an accredited laboratory should be conducted on both the water entering the treatment device and the finished water to verify that the treatment device is effective. Treatment devices lose their removal capacity through usage and time and need to be maintained and/or replaced. Consumers should verify the expected longevity of the components in their treatment device according to the manufacturer's recommendations and service it when required.
8.0 Kinetics and metabolism
The majority of uranium (>95%) that enters the body leaves via the feces rather than being absorbed (ATSDR, 2013). Uranium absorbed from the intestines, the lungs or skin enters the bloodstream and is then rapidly deposited in the tissues (mostly kidneys and bone), or excreted in the urine (Priest, 2001; Dublineau et al., 2005).
8.1 Absorption
Human and animal studies show that absorption of uranium via all routes is generally quite low (Arzuaga et al., 2015). Based on animal studies, the small intestine is the site of absorption following oral exposure, with little to no absorption occurring in the buccal cavity, stomach and large intestine (LaTouche et al., 1987; Dublineau et al. 2005; Konietzka, 2015).
In human studies, values for gastrointestinal (GI) absorption of uranium by adults calculated based on uranium excretion in urine and feces, varied from <0.1 to 6.5% (central estimate of 1 to 1.5%) (Hursh et al., 1969; Wrenn et al., 1989; Harduin et al., 1994; Leggett and Harrison, 1995; Karpas et al., 1998; Limson Zamora et al., 2003; Anke et al., 2009; Konietzka, 2015). Absorption showed a high inter- and intra-individual variation and was influenced by compound solubility with estimated GI absorption values of 0.2% for insoluble compounds and 2% for soluble hexavalent compounds (Hursh et al. 1969; Wrenn et al., 1985, 1989; Bhattacharya et al., 1989; Harduin et al., 1994; Leggett and Harrison, 1995; Karpas et al., 1998; Limson Zamora et al., 2003; Anke et al., 2009; ICRP, 2012; Konietzka, 2015). Fasting status also impacted absorption in adults. Overnight fasting in human subjects (2 fed and 4 fasted volunteers; not the same individuals who were tested fed then fasted) prior to uranium intake increased GI absorption from 0.5% (non-fasting) to approximately 2% (range of 0.5 to 5%) (Bhattacharyya et al., 1989).
Similarly, GI absorption in animals increased with the solubility of the uranium compound used, with the readily soluble uranium nitrate being the most easily absorbed compound (Sullivan, 1980b; Leggett and Harrison, 1995; Konietzka, 2015). The amount of uranium absorbed from the GI tract of animals ranged from 0.06 to 4.5% and was dependent on the species tested, increasing from rabbit (0.06%) to rat/mouse (<0.1%) to hamster (0.8%), to dog (1.5%), and to baboon (4.5%) (Sullivan, 1980a; Harrison and Stather, 1981; Larsen et al., 1984; Wrenn et al., 1985; La Touche et al., 1987). Fasting increased absorption values for baboons and mice from 0.51% and 0.069% (non-fasting) to 4.5% and 0.8% (fasted), respectively while fasting more than doubled GI absorption in Sprague-Dawley rats (fed 0.07% vs fasted 0.17%) (Sullivan et al., 1986; La Touche et al., 1987; Bhattacharyya et al., 1989; Konietzka, 2015). LaTouche et al. (1987) determined that Wistar rats also had higher uranium uptake via gavage following a 12 hour fast, but that feeding 2 hours after gavage also reduced GI absorption. The effect of iron and fasting on absorption was also studied. When fasted rats were gavaged with uranium nitrate alone or in combination with either ferric iron (oxidizing agent) or ferrous iron (reducing agent), absorption was significantly increased for fasted only and for fasted with ferric iron (100-fold increase) animals. This observed difference may be related to the oxidation state of uranium since the soluble U(VI) is more readily absorbed when compared to the insoluble U(IV) (Sullivan et al., 1986).
Human studies by Limson Zamora (2002a, 2002b, 2003) showed age had no effect on absorption via drinking water for adults (21 to 87 years of age) or teenagers (13 to 17 years) while Leggett and Harrison (1995) found age had no effect on absorption in subjects ≥ 5 years of age via the diet and/or drinking water. Both studies used urinary uranium content to calculate absorption. Data for absorption in infants and young children are sparse. Chen et al. (2010, 2011b), using bone ash samples, determined that the absorption value for infants was approximately 4 times higher than that of adults (9.3% compared to 2.1%) based on uranium while young children (1 to 7 years), and children (7 to 18 years) had absorption values of 5.0 and 3.0%, respectively. The Internal Commission on Radiological Protection (ICRP, 2012) estimated oral absorption values of 4% for infants and 2% for children ≥1 year of age based on adult human data and animal studies. ICRP's children's estimate is comparable to its adult absorption value of 2% for soluble uranium compounds (ICRP, 2012). Lower GI absorption related to aging is supported by animal studies that showed uptake from the gut progressively decreased with increasing age, approaching adult values by weaning and that neonatal rats gavaged with 233U-uranyl nitrate hexahydrate had increased absorption of uranium when compared to adult animals (Sullivan 1980a, 1980b, Sullivan et al., 1986; ICRP, 1995).
Human absorption values were independent of the atomic mass of uranium (233U vs 232U), exposure duration, total uranium intake and allocation of intake between food and water (National Research Council, 1999; Limson Zamora et al., 2002a, 2002b, 2003). However, a recent study (Larivière et al., 2013) using bone ash samples from seven regions in Western Canada suggested that the GI absorption value is higher when uranium intake is primarily via drinking water (3%) rather than via food consumption alone (0.9%). Limson Zamora et al. (2002a, 2002b and 2003) found that the sex of exposed individuals had no effect on absorption (with medians of 1.0% for females and 0.7% for males; p=0.13 in Kruskal-Wallis test) while Anke et al. (2009) saw minor sex differences in uranium absorption, with absorption values of 6.5% and 5.6% reported for females and males respectively. In animal studies, uranium absorption was independent of the atomic mass of uranium used, of the uranium's chemical speciation when ingested with water, of the animal's sex, and of the concentration of uranium in the diet (Sullivan, 1980a; Wrenn et al., 1985; Frelon et al., 2005; Konietzka, 2015). For rats, the rate of uranium absorption was also independent of the strain tested (Konietzka, 2015).
8.2 Distribution
Regardless of the route of entry, absorbed uranium can be found in all human tissues but is preferentially deposited in the kidneys and especially the bone within a few days after absorption (ATSDR, 2013). The amount of uranium deposited in tissues is proportional to the level of uranium intake and is strongly correlated with the uranium concentration in drinking water for bone, kidney and liver (Wrenn et al., 1985; Larivière et al., 2007, 2013; Dublineau et al., 2014; Poisson et al., 2014b). Bone is also the site of long-term uranium storage (Wrenn et al., 1985) and uranium is preferentially deposited on growing bone (Austin et al., 2000; Bourgeois et al., 2015) with bone growth resulting in the burial of uranium surface deposits (Austin et al., 2000). Animal studies indicate that the dose and length of exposure are important considerations for bone retention. Uranium from pellets chronically implanted in rats at high doses (up to 18 months) is continuously deposited in bone (skull and tibia) while uranium from pellets implanted at low doses reach a steady state similar to deposition in the kidneys (Pellmar et al., 1999). Subchronic studies in rabbits given uranium in drinking water for shorter durations of 28 or 91 d showed constant uranium retention that was independent of dose level (Tracy et al., 1992).Uranium is expected to have a slow-turnorver time in bone while uranium stored in soft tissues likely has a fairly rapid turnover time (Wrenn et al., 1985). Once uranium exposure has ceased, uranium is slowly released from bone and soft tissues (Orloff et al., 2004). Based on ICRP models, kidney burdens per unit intake were calculated for four age groups, ranging from infants to adults (Chen et al., 2004). Acute ingestion would result in an initial increase in uranium content in the kidney followed by a continuous decrease for all age groups. Chronic ingestion would initially cause a rapid increase in uranium kidney burden for all age groups that would gradually reach a steady level of 6.6% of daily uranium intake (Chen et al., 2004).
Based on ICRP's Reference Man, about 66% of the retained uranium is found in the bone, another 16% in the liver, 8% in the kidneys, and 10% in other tissues resulting in an estimated normal adult body burden of uranium of 90 µg (ATSDR, 2013). This model may overestimate uranium levels since experimental results have consistently reported lower values that ranged from 2 to 62 µg (Wrenn et al., 1985; Fisenne and Welford, 1986; National Research Council, 1999; Larivière et al., 2013). For example, Fisenne and Welford (1986) estimated that the human body contained 8 µg of uranium with mean values of 6.6 µg (86%) in the skeleton, 0.13 µg (2%) in kidneys, 0.36 µg (5%) in liver, and 0.50 µg (7%) in lung tissue. These variations have been attributed to individual dietary consumption patterns and uptake, local geology (Larivière et al., 2013), to the population being exposed (Dang 1995) and to subject age (Fisenne and Welford, 1986; Larivière et al., 2007).
Populations from different countries showed wide variations in uranium concentrations in similar organs, despite living in environments with comparable natural background levels and with no known additional exposure to uranium (Fisenne and Welford, 1986; Dang et al., 1995). Using tissue samples (lung, liver, kidney, skeleton, heart, muscle) collected from adult male traffic accident victims (aged 30-55 years, mean 42), Dang et al. (1995) reported that the uranium burden in an urban Indian (Bombay) population living in a normal background environment was greatest in the skeleton (2.80 µg), followed by muscle (1.2 µg), soft tissue (1.04 µg), lungs (0.98 µg), kidney (0.13 µg), liver (0.09 µg), and then the heart (0.04 µg). The geometric means for uranium concentrations (as fresh weight) in liver (0.08 ng/g) and muscle (0.058 ng/g) were much lower than those reported by other workers (0.19 to 0.25 ng/g and 0.19 to 0.47 ng/g respectively) studying American, British and Japanese populations, whereas concentrations in the heart and kidney tissues were comparable with other reports (Dang et al., 1995). Fisenne and Welford (1986) found that uranium concentrations in human bone varied by a factor of 10 for residents from the United Kingdom, Japan and New York City (3, 2, 0.3 µg/kg wet bone, respectively) although their daily dietary intake of uranium (1.0, 1.3, 1.5 µg per day respectively) differed only by a factor of 1.5. Sampling tissues (lung, liver, kidney and thoracic vertebrae and blood) from New York City accident victims (aged 14 to 73 year), also revealed that the tissue concentration of uranium increased with increasing age in the vertebrae and lung but not in the kidney or liver. For vertebrae, increases in uranium content were more significant above 50 years of age. No difference could be attributed to sex for any of the samples. Mean skeletal burden was estimated at 6.6 µg of uranium while the total body burden based on the tissues sampled was 8 µg (Fisenne and Welford, 1986).
Larivière et al. (2007) showed that uranium content of vertebrae varied both by location and age group (0–1 year, 1–10 year, 11–20 year, 21–30 year, 31–40 year, 41–50 year, 51–60 year and ≥61 year) using Canadian bone samples from two different locations in Canada (Winnipeg, MB and Regina, SK). Significantly higher uranium concentrations were seen in bone samples from Regina, particularly for the 0–1 year, 11–20 year and 21–30 year age groups, and were attributed to differences in the uranium content of the drinking water between the two locations (Larivière et al., 2007). Unlike the Fisenne and Welford (1986) study, the association of age with uranium concentration in the bones was not linear but linked to the calcium turn-over rate (Larivière et al., 2007). Uranium accumulation was highest in young individuals (<21 year) and represented periods of bone growth from child to adult. It decreased and levelled off for the middle age groups (21–60 year) and then increased in the ≥61 year group, representing bone decalcification related to osteoporosis (Larivière et al., 2007).
There is some evidence that accumulation of uranium in the bone may occur before birth in animals and humans with maternal uranium transferred through the placenta to the foetus resulting in an infant's initial body burden at birth being dependent on the mother's uptake (Wrenn et al., 1985; Chen et al., 2010). However, some studies did not report this finding. Experimental studies performed in pregnant rats did not show elevated uranium concentration in the foetus or embryo of dams exposed to 40 or 120 mg /L via drinking water (Legrand et al., 2015) although a significant dose- and time-dependent accumulation of uranium in pups was observed at postnatal days 0 to 21, suggesting pups were exposed via mother's milk (Legrand et al., 2015). Human studies have also indicated that exposure to maternal uranium levels for infants fed breast milk is possible (Wappelhorst et al., 2002; Ljung Bjorklund et al., 2012).
Tracy et al. (1992) reported that the fraction of uranium absorbed (administered via drinking water as uranyl nitrate hexahydrate) and deposited in the skeleton and kidney of rats was proportionally similar to humans with values for bone of 20 to 32 % (rat) vs 20 % (human) and for kidney of 7.2 to 45% (rat) vs 12 % (human). Retention half-times in the kidney were similar in rats (5-9 d) and humans (6 d). Retention half-times in bone of rats ranged from 40 to 180 d, as reported by Tracy et al., 1992 and would be equivalent to 1100 to 4980 d in human years (13.2 d rat = 365 d human (Sengupta, 2013)). Most organs of fasting animals contained substantially higher uranium than those found in animals fed normally, which is consistent with the higher absorption of uranium after fasting described previously. The most pronounced difference was in the kidneys of fasted animals, which contained approximately 8 times more uranium than the kidneys of non-fasted animals (Konietzka, 2015).
The accumulation and micro-distribution of uranium within the body and body tissues has been shown to change with time with chronic exposure or after exposure has ceased (Tracy et al., 1992; Paquet et al., 2006; Tessier et al., 2012). Male Sprague-Dawley rats given 40 mg uranyl nitrate/L of drinking water for 9 months showed 3 distinct peaks of whole-body uranium accumulation (equivalent to 182, 134 and 200 ng uranium/g respectively) at 95, 312 and 570 days. Other fluctuations in uranium content were seen in the femur and the kidneys with peaks at 95 and 570 days and, 220 and 311 days respectively (Paquet et al., 2006). Duration of exposure also influenced uranium distribution within organs, like the kidney. Male Sprague-Dawley were given 40 mg/L of uranyl nitrate (about 1 mg uranium per rat per day) in drinking water for 6, 9, 12 or 18 months (Tessier et al., 2012). An additional group of rats were dosed for 6 months and then allowed to recover for 3 months. Treatment for 12 months or less resulted in localized accumulation of uranium in the proximal tubules while distribution at 18 months was homogenously throughout the nephron. Distribution was attributed to long-term exposure and possibly to rat aging. Uranium was not detected in the renal cortex, in the proximal tubules or in the cortical collecting duct structures in the recovery group rats, suggesting the capacity to eliminate uranium once exposure had ceased (i.e., bioaccumulation may not be permanent) (Tessier et al., 2012). In a subchronic rabbit study, Tracy et al. (1992) gave 24 or 600 mg of uranyl nitrate hexahydrate/L (calculated as 1.24 to 1.33 mg uranium/kg bw per day or 36.7 to 41.7 mg uranium/kg bw per day) in drinking water to male rabbits for 91 days followed by a recovery period of 7 to 91 days. After 91 days of exposure, the mean uranium concentrations in the femur and kidney of the high dose group were 2.89 and 3.48 mg/kg respectively which decreased to 2.28 (femur) and 0.016 (kidney) mg/kg following a 91 recovery period.
The accumulation of uranium in the brain remains controversial, depending on the studies and the administration mode. After ingestion via drinking water, accumulation of uranium in whole brain was not clearly demonstrated (Houpert et al., 2005; Lestaevel et al., 2005) although some cerebral structures, such as striatum, cortex or hippocampus, preferentially accumulated more uranium (Dinocourt et al., 2015).
The results of uranium accumulation in gonads were contradictory depending on the species, the administration route, the uranium concentrations and duration of exposure. No accumulation of uranium was measured in ovaries of mice exposed to contaminated drinking water (Raymond-Wish et al., 2007; Arnault et al., 2008; Feugier et al., 2008). By contrast, a dose-dependence of uranium levels was observed in the offspring of rats exposed to uranium in their diet (Hao et al., 2012). Some animal studies observed an accumulation of uranium in testes (Paternain et al., 1989; Hao et al., 2012). Paternain et al. (1989) observed an accumulation of uranium in testes of Swiss mice which were administered 5, 10, and 25 mg/kg bw per day of uranyl acetate dehydrate before mating and up to 21 days post birth. The uranium content in testes of Wistar rats increased with the uranium concentration in food (Hao et al., 2012).
8.3 Metabolism
Regardless of the route of entry, once in the blood, uranium is predominantly present as the uranyl ion which mostly complexes with bicarbonate anions and with plasma proteins such as transferrin (Butterworth 1955; Adams and Spoor, 1974; Durbin and Wrenn, 1976; Ballou et al., 1986; Leggett, 1989; National Research Council, 1999; Keith et al., 2007; Basset et al., 2013; Arzuaga et al., 2015). It is not metabolized by the liver (Butterworth, 1955; Adams and Spoor, 1974; Durbin and Wrenn, 1976; Ballou et al., 1986; Leggett, 1989). The stability of the carbonate complex depends on the pH of the solution, which will differ in different parts of the body (National Research Council, 1988). The remaining uranyl ions may bind with smaller organic ions like citrate and maleate (Durbin and Wrenn, 1976) or become associated with erythrocytes (Wrenn et al., 1985; McDiarmid et al., 2012).
8.4 Excretion
In humans, over 95% of the uranium ingested via the diet or in drinking water passes through the digestive tract without being absorbed and is eliminated in the feces within 2 days (Hursh et al., 1969; Wrenn et al.,1988, 1989; Spencer et al.,1990).”Absorbed uranium is excreted via a two-phase model with half times in the kidney between 1 to 6 days for 99% of uranium and around 1500 days for the remainder (Keith et al., 2007). Once in the blood, the initial elimination of absorbed uranium is rapid, with most uranium being excreted in the urine within the first week following intake while less than 1% is excreted in the feces (Bleise et al., 2003; Keith et al., 2007; ATSDR, 2013). The highest uranium content in urine occurs 2 to 6 hours following acute ingestion (Karpas et al., 1998). The retention time in bone is around 11 days for the rapid phase and >70 days for the slow phase (Keith et al., 2007; ATSDR, 2013). An equilibrium between the amount of uranium excreted in the urine and the amount retained in the bone is reached within 1 to 40 days of continuous chronic uranium intake (Wrenn et al., 1985). In a population exposed to high uranium levels in drinking water (mean = 620 µg/L), uranium could still be detected in urine at levels exceeding the 95th percentile of the reference population up to 10 months after exposure ceased, although these were substantially lower (78%) than the levels seen at 2 to 4 months post-exposure. These elevated levels were attributed to uranium's slow release from bone and soft tissues and were expected to continue to decrease until a new equilibrium was reached (Orloff et al., 2004).
Excretion of uranium via the urine is independent of sex but dependent on age, increasing with increasing age (from 6 years) until age 60 when levels decline, although high levels seen following environmental exposure are still quite low when compared to occupationally exposed individuals (Werner et al., 1998; Al-Jundi et al., 2004; Tolmachev et al., 2006). Similar trends were seen in Poland with the highest excretion in adults (21–60 years) then declining after 60 years, with the lowest excretion in ages 3 to 20 years (Starosckiak and Rosiak, 2015). This trend was also seen in Eastern Europe (Priest and Thirlwall, 2001) and Bangladesh (population ranging from 8 to 88 years) (Berglund et al., 2011). Orloff et al. (2004) did not see a correlation between age and urine uranium content or between length of water consumption and urine uranium content in residents using a high uranium source for drinking water but a positive correlation was made between the uranium content of water and the uranium excreted. Conversely, Höllreigl et al. (2011) studying a Nigerian population exposed to low environmental levels of uranium found children (3 to < 15 years) excreted more uranium than adults (15 – 78 years).
Individual humans and animals were shown to have a high day-to-day variation in uranium excretion (Eidson et al., 1989; Werner et al., 1998). Uranium excretion was also influenced by fluctuations in acid-base balance of the urine, with increased alkalinity increasing urinary excretion while increasing acidity decreased it (McDiarmid et al., 2012).
Limited data indicate that uranium, like other heavy metals, can also be excreted through hair (0.37%) and nail growth (0.005%), although large variations in uranium concentrations in hair and nails were seen among individuals studied (Karpas et al., 2005).
9.0 Health effects
Although uranium is ubiquitous in the environment, it has no known essential metabolic function in humans or animals and is currently regarded as non-essential (Berlin and Rudell, 1986). Based on animal studies, the kidney is the major target organ for toxicity (see Sec. 9.2.2.1) although other target organs are possible (e.g., bone). Health effects from radiological forms and/or radioactive isotopes of uranium are addressed in a separate document (Health Canada, 2009).
9.1 Effects in humans
The epidemiological database is limited by meagre exposure estimates and inability to demonstrate causality (poor temporality and covariate assessments).
9.1.1 Acute toxicity
Available data on human health effects following acute oral exposure to uranium are limited. The observed outcomes vary, reflecting differences in doses, routes and concomitant exposures to other substances. For example, nausea, vomiting and diarrhea have been observed after ingestion of a single dose of 1 g of uranyl nitrate in drinking water by one individual (sex not specified) (Butterworth, 1955).
9.1.2 Chronic toxicity
In epidemiological studies, only kidney effects have been consistently found in humans, although recent studies have also shown effects in bone.
9.1.2.1 Kidney
In humans, chronic exposure to uranium via drinking water has been associated with elevated biomarkers of proximal tubule damage (i.e., increased urinary excretion of glucose, ions and low molecular weight proteins), and to a lesser extent, glomerular dysfunction (i.e., albuminuria). Although significant relationships between uranium exposure and biomarkers of kidney function have been observed, the clinical significance of the results remains unclear as most values were within normal levels. Moreover, many studies had poor exposure estimates, as they relied on urine samples and self-reported food and drink consumption, which can be severely biased and not representative of long-term levels.
In Nova Scotia, cross-sectional studies in two municipalities with a total of 324 residents exposed to drinking water containing variable amounts of naturally occurring uranium (up to 700 µg/L) from private wells exhibited no proteinuria or any symptoms or complaints (Moss et al., 1983; Moss, 1985). Individuals were stratified into exposure groups; however, there was no clear relationship between drinking water exposure and urinary concentration. A non-significant trend towards increasing excretion of urinary β2-microglobulin with increasing concentration of uranium in well water was observed, but the group with the highest uranium concentration failed to follow this trend (Moss et al., 1983; Moss, 1985). The raw data were not presented, selection bias was estimated to be high and there was no adjustment for confounders.
A cross-sectional study compared kidney outcomes between a high-exposure group –consuming drinking water with uranium concentrations of 1–781 µg/L (n = 30) and a low-exposure group (<1 µg/L, n = 20) (Limson Zamora et al., 1998). Most individuals with high uranium exposure through the drinking water came from Nova Scotia and had private wells, while most low exposure individuals were Ottawa residents supplied through the municipal distribution system. Individuals from the two cities were matched on sex and age, but pooled and divided between high and low exposure from the drinking water for the analysis. Total uranium intake from both water and food over a 3-day period was estimated. Glucose excretion and lactate dehydrogenase activity in female were significantly different between the two exposure groups (covariate adjustment was not specified). Some markers of tubular damage, such as glucose and β2-microglobulin excretion were positively correlated with uranium intake while others (alkaline phosphatase) were negatively correlated with uranium intake. Markers for glomerular functions (creatinine and protein) were not associated with uranium. The conclusions are limited by the study design which did not allow determination of temporality and the authors did not provide details on the adjustment for confounders. Also, there were large variations in the biomarker levels within each of the exposure groups, and no clear pattern was distinguishable by visually inspecting the plots showing biomarker levels in function of total uranium intake.
Another cross-sectional study was conducted to determine the association between uranium in the urine and effects on kidney functions of 54 Algonquin First Nations people of Kitigan Zibi in Quebec with different levels of uranium in their drinking water (Health Canada, 1998; Limson Zamora et al., 2009). There were 8 residents that had been exposed to levels between 111 and 178 µg/L; however, the installation of treatment devices had reduced these to 0.03-0.8 µg/L months before the study. Uranium excretion in the urine was positively correlated with urine volume, specific gravity, γ-glutamyl transferase (GGT) and β2-microglobulin (BMG) (weak to moderate correlations). The authors indicated these could reflect effects on renal proximal tubules; however, all BMG values were within normal range. Conversely, the authors found that the levels of alkaline phosphatase (ALP) and glucose excretion were not associated with increased uranium exposure. Moreover, the reliability of using uranium excreted in the urine as an indicator of past exposure based on its weak-to-moderate correlation (0.41) with a 15-year exposure estimate is questionable. The authors indicated that individuals moving and changing levels of uranium in the drinking water sources were very common. The risk of spurious associations was considered to be high.
A pilot cross-sectional study on urine albumin was conducted in three communities in Saskatchewan (n = 100) with mean uranium levels in the drinking water ranging from 0.71 to 19.6 μg/L (Mao et al., 1995). The cumulative index was calculated for each study participant as the product of the uranium concentration in drinking water, the number of cups of water consumed per day and the number of years lived at the current residence. A statistically significant association (p = 0.03) was observed between increased but normal levels of urine albumin and the uranium cumulative index, after adjusting for diabetic status, known risk factors for renal dysfunction, and age. However, the nature of the observed association is not clear as the participation rate was low (35.1%), exposure estimates did not consider previous places of residence or variations in concentration over time, and temporality cannot be established.
In Europe, Kurttio et al. (2002) conducted a cross-sectional study of 325 Finnish adults consuming drinking water with uranium concentration grouped as low (<10 µg/L), medium (10-100 µg/L) or high (>100 µg/L). Uranium in drinking water was significantly associated with increased calcium excretion while uranium in urine was associated with excretion of calcium and phosphate after adjusting for age, sex and body mass index (BMI); however, the values remained within normal levels and there was no observed dose-response. No association was observed with glucose, β2-microglobulin, creatinine, or albumin. Cumulative exposure was not associated with any outcomes, leading the authors to conclude that the observed changes in excretion are reversible. The relevance of the findings is unclear since no clear threshold was identified, important covariates were not adjusted for, and variations in the results were high. Kurttio et al. (2006a) further investigated uranium cytotoxic effects among a sample (n = 193) of the same cross-sectional study participants. The median exposure through drinking water was 25 µg/L, with a range of 0.001 to 1,500 µg/L, and an average duration of 16 years. No statistically significant associations were found between ten kidney biomarkers (e.g., urine ions, glucose, and creatinine) and uranium concentrations in the drinking water, urine, hair, toenails, or cumulative uranium intake, except between cumulative uranium intake and glucose clearance. A significant association was found between systolic and diastolic blood pressure after adjusting for smoking, age, BMI, and analgesics. However, the uranium exposure estimate was based on one water sample and no diet measurement was done.
A cross-sectional study comparing 301 adults with uranium drinking water concentration of 6.7 µg/L (<0.2 to 470 µg/L) with 153 controls (<0.2 µg/L) in Sweden found a small increase in tubular damage (i.e., β2-microglobulin, protein HC and kappa immunoglobulin chain), but not with female, or with cumulative uranium exposure, after adjusting for sex, age and smoking (Seldén et al., 2009). The authors indicated that there were no clear signs of nephrotoxicity from uranium in drinking water at levels recorded in this study and that the clinical relevance of these findings remains unclear. In Ireland, another cross-sectional study found no association between clearance of β2-microglobulin, retinol binding protein, albumin/creatinine ratio, and exposure to 120 to 142µg/L in drinking water (McDermott et al., 2005).
Magdo et al. (2007) reported a case of nephrotoxicity in a three-year-old girl consuming drinking water containing uranium in the range of 866 to 1,170 µg/L in rural Connecticut. The urine levels of β2-microglobulin were elevated in the youngest child of a family of seven (2 adults and 5 children) which might suggest a higher sensitivity to uranium for this young individual. No phosphate wasting or glycosuria was observed. The causative agent remains unclear since levels of arsenic, radon and radium were also elevated.
Studies conducted on children (6 – 12 years; n=100) from two Indian villages, one control and one near uranium mining (uranium concentrations/intakes were not measured) found a significant increase in the mean total protein: creatinine ratio (marker of renal disease) in urine (0.216 g/mg creatinine) compared to the normal (≤0.2 g/mg creatinine) reference range (Hedge et al., 2016) along with tooth effects (Kar et al., 2016; Shetty et al., 2016 – see section 9.1.2.2).
A case study from India reported a 12 year old boy with renal dysfunction (oliguria, uranium in urine 785.9 ng/d) related to ingestion of drinking water containing up to 224 µg/L of uranium (Chamberlain, 2009; Pannu et al., 2015).
War veterans with depleted uranium fragments have generally shown no associations with kidney outcomes (McDiarmid et al., 2004, 2007; Squibb and McDiarmid, 2006). For example, no clear signs of nephrotoxicity (urine biomarkers) or other health effects have been found between those with high versus those with low uranium concentration in the urine (≥0.1 vs. <0.1 µg/g creatinine) in a cohort of Gulf war veterans (n = 74) exposed to depleted uranium through retained fragments followed since 1993 (McDiarmid et al., 2009).
In conclusion, acute and chronic exposures to uranium species have been historically associated with renal damage, an endpoint that has generally been the focus of epidemiological studies on uranium. The most common associations observed between uranium exposures (measured in drinking water and urine) and human health were increased excretion of biomarkers of renal tubular dysfunction, such as β2-microglobulin and ions. A minority of studies have also shown associations with glomerulus bio-indicators with increased excretion of larger molecules (e.g., albumin). However, key endpoints need to be confirmed in more robust studies. In fact, considerable variability exists across the results and most observed associations were mild; some studies even reporting no associations with biomarkers such as glucose and β2-microglobulin. Moreover, the epidemiological literature is largely composed of studies observing correlations without considering covariates or reporting associations at a point in time. These do not allow reducing the risk of spurious relationships, determining whether the cause precedes the effect, or reliably quantifying exposures. Indeed, no studies identified a clear exposure threshold, and all have estimates that do not account for individual water consumption, changes in concentration with seasons, and cumulative dose over multiple years. Hence, there is a need to better the understanding of the nature of the relationship, and it would be premature to characterize a dose-response based on the present database.
9.1.2.2 Bone
Using the same study population as their 2002 study (described in section 9.1.2.1), Kurttio et al. (2005) conducted a study looking at bone biomarkers in adults (26 – 83 years) ingesting uranium via drinking water (median 27 µg/L; range 6–116 µg/L) for an average of 13 years. The results did not demonstrate a clear relationship between bone biomarkers and uranium exposure. Serum type I collagen carboxy-terminal telopeptide levels (marker for bone resorption) were the only biomarker associated with uranium exposure and this result was significant with a p-value of 0.05 in men only.
Two recent studies showed effects on tooth eruption and enamel defects (indicative disruption in bone deposition and resorption) related to uranium exposure. The first study comparing permanent tooth eruption in children (6 – 12 years; n=100) from two Indian villages, one control and one near a uranium mine (uranium concentrations/intakes were not measured) found a significant delay in tooth eruption (which involves bone remodeling – selective deposition and resorption of bone around the dental follicle) in the uranium exposed group (Shetty et al., 2016). A further study (Kar et al., 2016) found a significant proportion (82%) of children from villages near uranium mines (6 – 12 years; n=100) had enamel defects (which occur during tooth development) that were possibly linked to uranium exposure when compared with a control group from the same area but who were older than 20 years (i.e., not exposed during tooth development due to the timing of the mine opening). Neither study measured uranium exposure levels. Concurrent exposure to other chemicals (e.g., fluoride) likely occurred but was not measured.
The Pannu et al. (2015) case study cited in the previous section also reported chondroblastic type osteosarcoma in a 12 year old boy with renal dysfunction related to ingestion of drinking water containing up to 224 µg/L of uranium (Chamberlain, 2009; Pannu et al., 2015).
9.1.2.3 Other effects
In Kurttio et al. (2006a), previously described in Section 9.1.2.1, supine blood pressure was measured in men (n=95) and women (n=98) aged 18 to 85 years who drank water containing a median uranium concentration of 25 µg/L, with a range of 0.001 to 1,500 µg/L, and an average duration of 16 years. After adjusting for smoking, age, BMI, and analgesics, uranium exposure was associated with greater diastolic and systolic blood pressures but the effect was small and no clear hypertension was observed.
9.1.3 Carcinogenicity
The epidemiological evidence does not convincingly demonstrate an increased risk of cancer following uranium exposure (IARC, 1999, Lane et al., 2011). Small increases in cancer risk of lymphatic and hematopoietic tissues, bladder, stomach, lung, and brain have been reported in people working in the uranium enrichment and reprocessing facilities, millers and miners in France and the U.S. (Frome et al., 1990; Loomis and Wolf, 1996; McGeoghegan and Binks, 2000; Ritz et al., 2000; Boice et al., 2008; Vacquier, 2008; Guseva Canu et al., 2011). Other studies of uranium workers have reported no increase in cancer risk (Polednak and Frome, 1981; Waxweiler et al., 1983; Acquavella et al., 1985). All studies were retrospective, exploratory and hypothesis-generating in nature, not allowing causal determination. Although inhalation of a wide range of contaminants (e.g., vanadium, thorium, radium, lead, solvents) was common is these industries, co-exposures were not randomly distributed between groups and rarely adequately adjusted in the post-hoc statistical analysis. Uranium exposure was mostly estimated by modelling external radiation and through the inhalation route (oral exposure was never quantified and considered of lower magnitude), limiting the relevance of the results to the drinking water context. Individually, these studies had other limitations such as the absence of a dose-response relationship, the fact that workers exposed to the soluble species of uranium had no increase in cancer risk, the number of cases was low, many risk estimates were calculated, and the significance of the cancer effects was lost when compared with the general population.
Some environmental studies have observed an increase in cancer risk associated with the exposure to uranium in the drinking water. In Saskatchewan, a case-control study involving 88 cases of non-Hodgkin lymphoma found that cases had higher drinking water concentrations of uranium (mean = 10 µg/L) than controls (mean = 1.6 µg/L); however, this was an exploratory study in which cases were co-exposed to high levels of many contaminants of the drinking water (Witmans et al., 2008). Ecological studies in Germany and South Carolina have also found an association between leukemia, lung, colorectal, breast, kidney, prostate, and total cancers and higher levels of uranium in the drinking water (Wagner et al., 2011; Radespiel-Tröger and Meyer, 2013). The possibility to conclude on any effect based on these two studies is very limited since the results can only be applied to large geographical areas (inference to individual risk is not possible) and the exposure estimates were highly susceptible to bias.
In a cross-sectional study originally designed to address concerns over the incidence of cancer in the aboriginal community of Kitigan Zibi in Quebec (n = 54), which has had high levels of uranium in the drinking water, no increase in cancer risk was found in association with the levels in the urine (Limson Zamora et al., 2009). The residents had historically been exposed to a wide range of uranium in the drinking water (up to 1418 µg/L). In two case-cohort studies and one cross-sectional study in Finland (Auvinen et al., 2002, Auvinen et al., 2005; Kurttio et al., 2006b), leukemia, stomach, bladder, and kidney cancers were not associated with uranium in the drinking water (median = 10 µg/L). A case-control study in Nevada of 16 cases of leukemia and 100 controls found no significant differences in uranium concentration in wells between cases versus controls (i.e., uranium drinking concentrations in families with a child who had leukemia did not differ significantly from non-case families) (Seiler, 2004).
In conclusion, although some studies have found an association with cancer of different tissues, the epidemiological database on the cancer risk of uranium is weak and equivocal, and does not enable any conclusions with regard to carcinogenicity.
9.1.4 Developmental and reproductive toxicity
Studies in uranium miners have failed to find any consistent developmental or reproductive effects of uranium exposure (Müller and Ruzicka-Jaroslav Bakstein, 1967; Waxweiler et al., 1981b; Wiese and Skipper, 1986).
9.2 Effects on experimental animals
Based on animal experiments, uranium toxicity varies greatly with route and duration of exposure, species tested, animal age and sex, dose and the uranium compound used, with the more soluble compounds exhibiting greater toxicity (Maynard and Hodge, 1949; Orcutt, 1949; de Rey et al., 1983; Pelayo et al., 1983; Kathren and Burklin, 2008; Hamid, 2012). The main target organ for toxicity in animals exposed to high concentrations of uranium is the kidneys (Maynard and Hodge, 1949; Tannenbaum and Silverstone, 1951). Other effects have been seen in bone (Arzuaga et al., 2015), liver (Goel et al., 1979), brain (Bellés et al., 2005; Briner and Murray, 2005; Lestaevel et al., 2005) and the reproductive system (Feugier et al., 2008; Arnault et al., 2008; Kundt et al., 2009).
9.2.1 Acute toxicity
Animals exposed to acute, high doses of uranium exhibited increased urinary output, proteinuria, kidney damage, mild liver injury and death (Orcutt, 1949; Domingo et al., 1987). Oral LD50 values of 204 and 242 mg uranyl acetate/kg bw (calculated as114 and 136 mg of uranium/kg bw) are available for gavaged male Sprague-Dawley rats and male Swiss mice, respectively (Domingo et al., 1987).
Statistically significant changes were seen in endochondrial ossification (decreased cartilage growth and metaphyseal bone volume; increased resorption of metaphyseal bone with decreased formation and rest areas in metaphyseal bone) in the femurs of mice given a single gavage lethal dose of 350 mg uranyl nitrate/kg bw (Bozal et al., 2005).
9.2.2 Short-term and subchronic exposure
In animal studies, effects following short-term oral exposure varied with the species, strain and sex of the animals tested and the solubility and dose of the uranium compound used as well as the length of exposure (Maynard and Hodge, 1949; Tannenbaum and Silverstone, 1951; Hamid, 2012). Uranium was shown to preferentially target the kidneys and nervous system in a number of studies (Maynard and Hodge, 1949; Tannenbaum and Silverstone, 1951; Ortega et al., 1989; Gilman et al., 1998a, 1998b; Bellés et al., 2005; Lestaevel et al., 2005). Hepatic effects, hematological effects, alterations of the immune system, changes to gene and protein expression and bone growth effects were also seen (Tannenbaum and Silverstone, 1951; Goel et al., 1979; Ortega et al., 1989; Gilman et al., 1998a; Pujadas Biji et al., 2003; Pujadas Biji and Ubios, 2007; Hao et al., 2013). No signs of overt clinical toxicity were reported in the majority of studies (Ortega et al., 1989; Gilman et al., 1998a). In studies reporting clinical toxicity, the effects included decreased body weight gain or increased weight loss in rats, rabbits and mice (Maynard and Hodge, 1949; Tannenbaum and Silverstone, 1951; Gilman et al., 1998b). In both rats and rabbits, males were the more sensitive sex (Maynard et al., 1953; Gilman et al., 1998a, 1998b) while adult rats and mice were more sensitive than weanling animals (Maynard and Hodge, 1949; Tannenbaum and Silverstone, 1951).
9.2.2.1 Kidney
A number of drinking water studies in animals have identified the kidney as the primary target for uranium toxicity (Maynard and Hodge, 1949; Tannenbaum and Silverstone, 1951; Ortega et al., 1989; Gilman et al., 1998a,1998b). Reported effects varied in intensity, ranging from microscopic lesions to necrosis and included changes in blood chemistry, urinary markers and gene expression relating to kidney function, red blood cell counts, and iron metabolism, although some studies found no marked kidney effects even at doses of 600 mg/L of uranyl nitrate (equivalent to 40 mg uranium /kg bw per day) given for up to 9 months (Maynard and Hodge, 1949; Tannenbaum and Silverstone, 1951; Goel et al., 1979; Ortega et al., 1989; Gilman et al., 1998a, 1998b; Taulan, 2004; Donnadieu-Claraz et al., 2007; Berradi et al., 2008; Poisson et al., 2014b). Changing the length of exposure also had inconsistent results on histopathological effects in the kidney. Comparing 3 month and 9 month exposures in rats, Poisson et al. (2014b) did not observe differences in kidney histopathology related to exposure time or dose while Hamid (2012) observed more pronounced renal histopathological findings in rats gavaged daily with 75 mg/kg bw of uranyl nitrate (35 mg uranium /kg bw per day) following 60 days of dosing compared to 30 days. Although gene expression (Kim-1, Timp-1, lipocalin-2, OPN, clusterin and vimentin) changes in kidneys were not associated with histopathological changes in the kidney, Rached et al. (2008) demonstrated that these changes precede alterations in clinical indicators of impaired kidney function and were correlated with progressive histopathological changes.
Gilman et al. (1998a, 1998b) reported a lowest-observed-adverse-effect-level (LOAEL) of 0.96 mg/L of uranyl nitrate hexahydrate in rats (equivalent to 0.09 mg uranium/kg bw per day in females and 0.06 mg uranium/kg bw per day in males) and male rabbits (equivalent to 0.05 mg uranium/kg bw per day) based on kidney effects following 91 days of exposure to 0 to 600 mg/L of uranyl nitrate hexahydrate in drinking water. Male rabbits were not pathogen-free which may explain the higher LOAEL of 4.8 mg uranyl nitrate hexahydrate/L (equivalent to 0.49 mg uranium/kg bw per day) reported for female rabbits (Gilman et al., 1998b). An additional rabbit study (Gilman et al., 1998c) using pathogen-free males showed less severe kidney effects, with a LOAEL estimated at ≤24 mg/L of uranyl nitrate hexahydrate (1.36 mg uranium/kg bw per day). Ortega et al. (1989) reported a no-observed-adverse-effect-level (NOAEL) of 2 mg uranyl acetate dihydrate kg bw per day (equivalent to 1 mg uranium/kg bw per day) for male Sprague-Dawley rats based on an increase in total plasma protein levels although changes in kidney histopathology were only seen at the highest dose used (16 mg uranyl acetate dihydrate/kg bw per day; equivalent to 9 mg uranium/kg bw per day).
In a study by Gilman et al. (1998a), weanling Sprague-Dawley rats (15/sex/group) drank water containing uranyl nitrate hexahydrate at <0.001 (control), 0.96, 4.8, 24, 120 or 600 mg/L (equivalent to <0.0001, 0.06, 0.31, 1.52, 7.54 and 36.73 mg uranium/kg bw per day in males and <0.0001, 0.09, 0.42, 2.01, 9.98 and 53.56 mg uranium/kg bw per day in females) for 91 days. No clinical effects or differences in intake of food and water were observed. Histopathological changes were seen in both sexes in the thyroid, spleen, kidney and liver, although liver effects were considered adaptive. Thyroid lesions were statistically significant at ≥4.8 and ≥24 mg uranyl nitrate hexahydrate/L in males and females respectively but were not considered specific to uranium treatment by the authors. Sinus hyperplasia of the spleen was seen in both sexes in a dose-dependent manner but was only significant at the highest dose. Statistically significant treatment-related kidney lesions were seen at all doses and in both sexes but were generally less severe in females. In males, significant renal effects were observed in tubules (dilation, apical displacement, and vesiculation of tubular nuclei and cytoplasmic vacuolation and degranulation) at the lowest exposure level. Other lesions, including glomerular adhesions and focal tubular degranulation, became significantly different above 4.8 mg uranyl nitrate hexahydrate/L. In females, the most important kidney changes were sclerosis of glomerular capsules and reticulin sclerosis of tubular basement membranes and interstitial scarring, both of which are unrepairable lesions that occurred at the lowest exposure level. Based on kidney effects, the LOAEL was 0.96 mg uranyl nitrate hexahydrate/L (equivalent to 0.09 mg uranium/kg bw per day in females and 0.06 mg uranium/kg bw per day in males)(Gilman et al., 1998a).
Similar results were seen in another drinking water study conducted by Gilman et al. (1998b) using weanling New Zealand white rabbits given the same doses of uranyl nitrate hexahydrate in drinking water (males given <0.001 (controls), 0.96, 4.8, 24, 120 or 600 mg/L and equivalent to 0, 0.05, 0.20, 0.88, 4.82 and 28.7 mg uranium/kg bw per day; females given <0.001 (controls), 4.8, 24 or 600 mg uranyl nitrate hexahydrate/L and equivalent to 0, 0.49, 1.32 and 43.02 mg uranium/kg bw per day) for 91 days. Histopathological changes were observed in the kidney (primarily the proximal tubules), liver, thyroid and aorta (not dose-dependent) in both males and females in all doses tested but were less severe in females than in males despite females consuming on average 65% more water than the males. Males were not free of infection which may have affected the severity of effects seen. Based on kidney effects (foci of cytoplasmic vacuolation in proximal renal tubular epithelium, vesiculation and pyknosis of tubular nuclei), the LOAEL was the lowest dose tested for each sex - 0.96 mg uranyl nitrate hexahydrate/L (equivalent to 0.05 mg uranium/kg bw per day) for males and 4.8 mg uranyl nitrate hexahydrate/L (equivalent to 0.49 mg uranium/kg bw per day) for females.
In a follow-up study (Gilman et al., 1998c) to assess the reversibility of the kidney effects previously observed (Gilman et al., 1998b), groups of 5 to 8 pathogen-free male New Zealand white rabbits were given <0.001 (control), 24 or 600 mg uranyl nitrate hexahydrate/L (equivalent to 0, 1.36 and 40.98 mg uranium/kg bw per day) in drinking water for 91 days, with a recovery period of up to 91 days. Minor histopathological lesions were seen in the liver, thyroid and aorta. In the kidney, focal dilation of the renal proximal tubules was observed at both doses. Tubular injury with degenerative nuclear changes, cytoplasmic vacuolation and tubular dilation was observed in the high-dose group and was still present after a 91-day recovery period. The presence of sclerotic changes in the tubular basement membranes and renal interstitium persisted during the recovery period and most likely represents a permanent injury (McDonald-Taylor et al., 1992, 1997; Gilman et al., 1998c). Although the histopathological changes observed in this study were similar to those noted in the female rabbits of the previous study, males in the present study did not respond as dramatically as in the earlier study (Gilman et al., 1998b) potentially indicating that pathogen-free rabbits may be less sensitive than infected animals to the effects of the uranyl ion in drinking water. Based on the histopathological data in the kidney, a LOAEL for the male New Zealand rabbits in this study is estimated at ≤1.36 mg uranium/kg bw per day (Gilman et al., 1998c).
Using a similar dose range as the Gilman et al. (1998a) rat study, Poisson et al. (2014b) gave male Sprague-Dawley rats (age not specified) drinking water containing 0, 1, 40, 120, or 400 mg/L of uranyl nitrate for 3 months or 0, 1, 40, 120, or 600 mg/L of uranyl nitrate for 9 months. The uranium content in renal tissue of treated animals was proportional to its concentration in the drinking water. Changes to kidney histopathology (glomerular lesions, tubular regeneration, tubular dilation, interstitial inflammation and fibrosis) were comparable in intensity and incidence across all groups, including the control, and were not dose- or time- dependent. However, both duration of and exposure to uranium affected the antioxidant system in the renal cortex. In the kidney, lipid peroxidation levels (measured by thiobarbituric acid-reactive substances - TBARS) statistically decreased after 9 months at ≥120 mg/L while alterations in glutathione metabolism were seen at 3 months in reduced glutathione (GSH), glutathione peroxidase (GPx) and glutathione-S-transferase (GST) levels but did not persist to 9 months except for a dose-dependent increase in GSH levels at ≥40 mg/L. Proteinuria increased significantly (p<0.05) from baseline after 3 and 9 months for the control groups and the 40 and 120 mg/L groups but not in the lowest and highest dose groups. The authors attributed these changes in protein levels to animal aging as no difference was seen among groups at either time point. A LOEL of 40 mg/L of uranyl nitrate, based on increased GSH levels, could be estimated. (Poisson et al., 2014b).
Taulan et al. (2004) also showed oxidative stress in the kidneys as well as changes in gene expression in male C57BL/6J mice given 0, 80, or 160 mg uranyl nitrate/L in drinking water for 4 months. No gross pathology was found in the kidneys although their uranium content increased in a dose-dependent manner. Alterations in expression were seen in over 200 genes (mostly up-regulation) that were responsible for oxidative response, encoding ribosomal proteins, cellular metabolism, signal transduction and solute transport. There was a significant, dose-dependent increase in hydrogen peroxide production in the kidney that was accompanied by an increase in mRNA levels for copper-zinc superoxide dismutase (SOD) and GPx. Berradi et al. (2008) administered 40 mg/L as uranyl nitrate hexahydrate (2.4 mgU/kg/d as calculated by ATSDR, 2013) in drinking water to 3-month old male Sprague-Dawley rats for 9 months and observed an increase in the severity of tubule-interstitial lesions but not in their frequency. This observation was accompanied by a 90% decrease in mRNA levels of the renoprotective gene erythropoietin receptor (EPOR) and a 12-fold increase in ceruloplasmin (CP) mRNA which is also renoprotective.
Induced oxidative stress and depletion of the antioxidant defense system was seen in a study by Linares et al. (2006) in which sexually mature male Sprague-Dawley rats given 0, 10, 20 and 40 mg uranyl acetate dihydrate/kg bw per day in drinking water for 3 months showed a statistically significant, dose-dependent increase in SOD activity in kidneys at all doses. TBARS and oxidized glutathione (GSSG) levels increased with increasing renal uranium concentration. Histopathology of the kidney included dilated capillaries with prominent endothelium in all treated groups. No correlation between uranium concentration and GSH levels was seen in the kidneys. A LOAEL of 10 mg uranyl acetate dihydrate/kg bw per day (calculated as 5.6 mg uranium/kg bw per day) was estimated based on kidney histopathology.
No overt nephrotoxicity was reported in a comprehensive 9 month drinking water study (Dublineau et al., 2014) that dosed 8-week old male Sprague-Dawley rats (10/group) with 0.2, 2, 5, 10, 20, 40, or 120 mg/L depleted uranyl nitrate hexahydrate (0.009, 0.09, 0.23, 0.45, 0.9, 1.8, or 5.4 mg uranium/kg bw per day). No changes in plasma parameters, including renal function and iron metabolism were observed. Histopathological examination of the kidney did not reveal any tubular necrosis regardless of dose. Glomerular lesions, scattered cysts and limited tubulointerstitial lesions (mostly consisting of multifocal inflammatory cell infiltrates) were limited and seen in all rats, including controls. Tubular regeneration and tubular inflammation were both slightly, but not statistically significantly, increased at 10 and 40 mg/L (0.45 and 1.8 mg uranium/kg bw per day), and 0.2, 10 and 40 mg/L (0.009, 0.45 and 1.8 mg uranium/kg bw per day) respectively. These increases were very limited and were not observed at the 120 mg/L dose. Xenobiotic metabolism in the kidney was assessed using major enzymes (XME) and proteins related to its three phases. Only a dose-dependent increase in ST1A1 gene expression that was statistically significant at all doses was observed. The authors proposed a no-observed-adverse-effect level (NOAEL) of >120 mg uranyl nitrate hexahydrate/L (>5.4 mg uranium/kg bw per day) since alterations in XME were seen as low as 10 mg/L (0.45 mg uranium/kg bw per day) in the kidney and were not accompanied by signs of tubular regeneration and inflammation.
Gueguen et al. (2014) provided 3-month old male Sprague-Dawley rats (n=12/group) with either 40 mg/L of uranyl nitrate (1.8 mg uranium/kg bw per day) in drinking water for 0, 1, 3, 6, 9 or 18 months (experiment 1) or with 0, 10, 40 and 120 mg uranyl nitrate/L (equivalent to 0.45, 1.8 5.4 mg uranium/kg bw per day) for 9 months (experiment 2) in drinking water. No effect was seen on food or water intake, final body weight or on kidney or liver weights in either study. As well, no changes were seen in plasma biomarkers for kidney (creatinine, urea) or for liver (transaminase, alkaline phosphatase) function or in kidney and liver histopathology. In experiment 1, changes were noted in gene expression of XME in the liver and kidney, including CYP3A enzymes, and were significant at 6 and 9 months but not at 1, 3 or 18 months. The lack of response at 18 months was attributed to aging. In experiment 2, uranium accumulated in the kidneys and liver in a dose-dependent way. The most significant changes occurred: in the liver at 120 mg/L consisting of decreased CYP3A enzyme activity correlated with decreases in its gene and protein expression; and in the kidneys at ≥10 mg/L consisting of an increase in gene expression of ST1A1. An LOEL of <10 mg/L (<0.45 mg uranium/kg bw per day) could be determined based on minor changes in gene expression.
Changes in plasma parameters relating to kidney function, including total protein were seen in male Sprague-Dawley rats (8/dose; age not provided) given 0, 2, 4, 8, or 16 mg/kg bw per day of uranyl acetate dihydrate (equivalent to 0, 1, 2, 4 or 9 mg uranium/kg bw per day) in drinking water sweetened with sugar (amount not specified) for 4 weeks. Controls were given sweetened water only. Kidneys, thyroid, bone and muscle tissues accumulated uranium in a dose-dependent way but comparison with the control animals was not done (control rats not tested). Histopathological effects (congestion of liver, spleen, kidney and moderate increase in proximal tubule epithelial cell lysosomal content) were seen only at the 16 mg/kg bw per day group. In plasma, increases in hematological parameters were seen at 8 mg/kg bw per day (MCHC – mean corpuscular hemoglobin concentration) and 16 mg/kg bw per day (hematocrit, erythrocytes, hemoglobin, MCHC). Clinical chemistry parameters showed both increases at ≥2 mg/kg bw per day (total protein) and at 4 mg/kg bw per day (glucose) and decreases at mg/kg bw per day (GOT – glutamic oxaloacetic transaminase, GPT – glutamic pyearuvic transaminase). The authors considered 2 mg/kg bw per day of uranyl acetate dihydrate (1 mg uranium/kg bw per day) to be the NOAEL based on an increase in plasma total protein levels (Ortega et al., 1989) although a statistically significant increase in total protein was seen at this dose.
Renal anemia is an early symptom of chronic kidney disease (Berradi et al., 2008) and uranium may affect iron homeostasis within the kidney (Donnadieu-Claraz et al., 2007). In contrast to the findings in Ortega et al. (1989), Berradi et al. (2008) and Donnadieu-Claraz et al. (2007) observed iron disorders in rats following chronic exposure to uranium.
Three-month old male Sprague-Dawley rats were given 0 mg/L (n=7) or 40 mg/L (n=8) of depleted uranium (DU as uranyl nitrate hexahydrate) (2.4 mg uranium/kg bw per day calculated by ATSDR, 2013) in drinking water for 9 months. A 20% decrease in red blood cell (RBC) counts, hematocrit and hemoglobin levels was seen in the dosed group although treatment had no effect on iron levels and iron total and unsaturated binding capacities. No change in the total iron content of the kidney was observed but its distribution was modified. There was no change in blood cytokine levels. Uranium did not have a significant effect on general hematopoiesis or erythropoiesis. Treatment did not affect the frequency of tubule-interstitial lesions but it did increase their severity although no functional changes, as measured by creatinine and urea blood levels, were observed. Gene expression of CP, a superoxide scavenger, was 12 times higher in treated rats while splenic mRNA levels of DMT1 (divalent metal transporter 1) were increased threefold. DU induced renal deterioration that in turn decreased RBC count (Berradi et al., 2008).
Donnadieu-Claraz et al. (2007) reported iron accumulation in kidneys of male Sprague-Dawley rats (10 weeks old) given 40 mg/L of uranyl nitrate (2.7 mg uranium/kg bw per day) in drinking water for 6, 9, 12 or 18 months. No difference in food or water consumption or in body weights was seen between the control and treated groups. Accumulation of uranium in the kidneys was highest at 32 days (220 ng/g) and at 570 days (300 ng/g) and ranged from 70 to 100 ng/g between these two peaks. A large variation in urinary uranium excretion was seen among exposed rats, although average excretion values peaked at 0.38 µg/mL of urine around 200 days. Significant changes in proximal tubular cells appeared at 6 to 9 months of exposure. By 12 to 18 months, normalization of the epithelia could be seen although debris was still present in the tubule lumen. Increases in both the number of intracellular vesicles and the presence of clusters of small iron oxide granules within vesicles could be seen starting at 6 months and increased in number with exposure length. The authors suggested that uranium exposure may affect iron homeostasis in the kidneys. Although iron is an important component of cytochromes, certain enzymes and oxygen-binding molecules, it can generate reactive oxygen species via the Fenton reaction and damage tissue (Ray et al., 2012).
Other studies (Rouas et al., 2011; Grison et al., 2013; Poisson et al., 2014a) using only 40 mg/L of uranyl nitrate hexahydrate (average dose of approximately 2.7 mg uranium/kg bw per day) administered in drinking water for 9 months to male Sprague-Dawley rats did not show any effects on kidney function (diuresis, creatinine clearance, creatinine, urea, total protein), histology or novel markers of kidney toxicity (such as KIM-1, osteopontin and kallikrein), with the exception of a decrease in creatinine clearance reported by Poisson et al. (2014a) and increases in plasma AST and creatinine and in urine potassium and sodium levels (Grison et al., 2013). Using metabolomics, Grison et al. (2013) was able to differentiate between treated and untreated animals, particularly using N1-methylnicotinamide which is a potential early indicator of nephrotoxicity, and concluded that the absence of overt toxicity does not necessarily imply a lack of biological effects since changes in metabolite levels, such as N1-methylnicotinamide, were noted. According to Devarajan (2010), functional changes are inherently delayed due to “renal reserve” or the ability of the kidney to maintain its glomerular filtration rate (GFR) via hyperfiltration and compensatory hypertrophy of the remaining healthy nephrons despite progressive destruction of nephrons. This renal reserve allows for continued clearance of plasma solutes, so that the plasma markers (creatinine and urea) show significant increases only after about 50% of the GFR has been lost. In addition, the enhanced tubular secretion of creatinine that is characteristically encountered at lower rates of GFR results in an overestimation of renal function (Devarajen, 2010).
No reports are available of studies where toxic responses of young animals to uranium were directly compared to those of adults. Three studies by Maynard et al. (1953) evaluated age-related differences in uranyl nitrate toxicity in rats aged 17 days to 6 months exposed to 2% uranyl nitrate in the diet for 30 days; in rats aged 1, 2, 3, or 6 months exposed to 2% uranyl nitrate in the diet for 24 hours followed by a 30-day observation period; and in rats aged 21 days to 6 months receiving a single intraperitoneal (i.p.) injection of 128 (males) or 200 (females) mg/kg uranyl nitrate hexahydrate. Two of the three studies found age-related increases in mortality. In the 30-day dietary exposure study, >75% of the 17- and 21-day-old animals died during the study, <10% of the 28-day-old animals died, and >50% of the 2-, 3-, 4-, 5-, and 6-month-old animals died. Data provided on body weight and food intake were insufficient to calculate daily doses. The 1-day dietary study found a similar pattern of mortality. Mortality increased with age; 1% (males) and 3% (females) of the 1-month-old rats died compared to 8% (males) and 16% (females) of the 6-month-old rats. Following a single intraperitoneal dose, the mortality rates were 36, 11, 8, 18, 24, and 19% males and 24, 18, 8, 23.5, 41, 22, and 52% of the females aged 21 days, 1 month, 2 months, 3 months, 4 months, 5 months, and 6 months, respectively. The differences in mortality may be due to age-related toxicokinetic differences, such as changes in absorption efficiency, skeletal development, or kidney development (ATSDR, 2013).
From early studies, it appears that tolerance may develop in the kidney following repeat dosing, so that much larger doses may be needed to produce the same effect seen with the initial small dose (Yuile, 1973; Durbin and Wrenn, 1976; Campbell, 1985). Rats exposed to chronic low doses of uranium for 1 or 2 years exhibited mild renal injury accompanied by transient changes in urinary and plasma biochemical markers suggesting that at low exposures the renal tubular epithelium is regenerated and continued exposure does not result in more severe effects (Maynard and Hodge, 1949; Maynard et al., 1953). In a serial study, rats exposed to 170 mg uranium/kg bw per day as uranyl nitrate in the diet had regeneration of the renal tubular epithelium after 2 weeks of exposure (Maynard et al. 1953). Continued exposure did not result in progression of renal damage so that the renal tubules in rats exposed for 2 weeks were similar to those exposed for 1 year (Maynard et al. 1953). Additionally, a 2 year exposure to 170 mg uranium/kg bw per day did not result in any further damage to the kidneys (Maynard and Hodge, 1949; Maynard et al., 1953). In contrast, regeneration was observed in the first month of the exposure to 660 mg uranium/kg bw per day, however, with continued exposure, tubular atrophy was observed at 6–8 weeks. The severity of the atrophy and the areas of the kidney affected by uranium increased with duration. However, at higher doses, the capacity to regenerate the renal tubular epithelium is exceeded and tubular atrophy is observed (Maynard et al., 1953). The return to normal values for the biochemical parameters during chronic exposure may reflect a kind of acquired tolerance to uranium associated with structural changes in the luminal surfaces of regenerated kidney tubule cells. This tolerance does not, however, prevent chronic damage to the kidney, as the regenerated cells are quite different; although histopathologically it may appear that the repair process is well advanced, the urinary biochemical changes return to normal only slowly (Leggett, 1989).
Uranium tolerant animals have been shown to have diminished urinary alkaline phosphatase (Yuile, 1973) and decreased glomerular filtrate rate (Morrow et al., 1982). Proximal tubule cells were particularly affected by uranium toxicity. Alterations causing thickening of the glomerular basement membrane of the kidney, which results from the storage of uranium in the kidney, can be prolonged and severe enough to cause permanent damage (McDonald-Taylor et al., 1992). Persistent ultrastructural changes in the proximal tubules of rabbits have also been reported to be associated with the kidney's ability to store uranium (McDonald-Taylor et al., 1997). Cell damage in the proximal tubules was significantly more severe in animals allowed up to a 91-day recovery period than in animals killed at the end of the exposure period (Gilman et al., 1998c). Acquired tolerance should therefore not be considered as a practical method of protection against uranium intoxication.
9.2.2.2 Central nervous system
Once in the bloodstream, uranium can cross the blood-brain barrier, preferentially accumulating in the frontal and temporal cerebral cortex, and the hippocampus regions of the brain although not all studies have shown dose-dependent accumulation (Lemercier et al., 2003; Bussy et al., 2006; Paquet et al., 2006; Bensoussan et al., 2009; Dublineau et al., 2014; Ibanez et al., 2014). Linares et al. (2007) reported a dose-dependent increase in uranium content in brains of male Sprague-Dawley rats given 10, 20 or 40 mg uranyl nitrate dihydrate/kg bw per day (calculated as 6, 11, or 22 mg uranium/kg bw per day) in drinking water for 3 months, while Bellés et al. (2005), using a similar protocol, found that uranium accumulation in the brains of male Sprague-Dawley rats was not dose-dependent since the lowest level was seen in the middle dose group (11 mg uranium/kg bw per day). Paquet et al. (2006) found that the uranium content in the brains of adult male Sprague-Dawley rats given 40 mg uranium/L fluctuated over time, with the uranium in whole brain decreasing over 32 to 186 d from 3 to 0.07 ng uranium/g brain tissue, then increasing gradually until 570 d to about 1 ng uranium/g brain tissue.
Most studies involving ingestion via drinking water showed no overt signs of toxicity (Briner and Murray, 2005) and in those that did, the effects were mild (e.g., decreased body weight gain; Bussy et al., 2006). Neurological effects were minor, inconsistent, and sometimes contradictory, often lacking a dose-response relationship. They included: behavioural changes in the open field test in male rats dosed for 3 months with 10 and 20 mg uranyl nitrate dihydrate/kg bw per day in drinking water but not with 40 mg uranyl nitrate dihydrate/kg bw per day (Bellés et al., 2005) and in male (75 and 150 uranyl nitrate dihydrate/kg bw per day) and female (150 uranyl nitrate dihydrate/kg bw per day) rats dosed for 6 months and in males (150 uranyl nitrate dihydrate/kg bw per day) dosed for 2 weeks (Briner and Murray, 2005); and disruptions to the sleep-wake cycle in male rats given 40 mg U/L in drinking water for 90 days (Lestaevel et al., 2005). Changes in the monoaminergic systems were seen when a dose of 40 mg/L of uranyl nitrate hexahydrate (equivalent to 4 to 1.5 mg/kg bw per day) in drinking water was given to rats. These changes were more pronounced at 9 months when compared to 6 and 1.5 months and were accompanied by significant uranium accumulation in the striatum (at 1.5 and 9 months), the hippocampus and frontal cortex (at 9 months) (Bussy et al., 2006). Changes were also seen in lipid oxidation levels in the brain (Briner and Murray, 2005) and in the cholinergic system (Bensoussan et al., 2009) as well as increased oxidative stress (Linares et al., 2007). Most studies did not report histological changes in the brain, although Kelada et al. (2008) did report focal degenerative changes and ultrastructural alterations in the cerebral cortex and increased staining of neuroglial cells in rats gavaged with 60 µg/kg bw of Uranyl acetate dihydrate for 90 days. In vitro studies did not find any significant cytotoxicity in either rat brain endothelial cells (Dobson et al., 2006) or primary rat cortical neurons and only minimal effects on thiol metabolite levels, redox potential and lipid peroxidation (Jiang et al., 2007).
9.2.2.3 Immune system
Alterations in immune function have been studied in 3-week old Kunming mice fed diets containing 0, 3, 30, or 300 mg uranyl nitrate hexahydrate/kg of feed (equivalent to 0, 0.4, 4, 40 mg uranium/kg bw per day) for 4 months (Hao et al., 2013a). Concentration dependent increases in the proliferation of splenic B lymphocytes and in the levels of splenic cell interleukins IL-4 and IL-10 were seen at ≥ 3 mg/kg. The proliferation of splenic T-lymphocytes was decreased at ≥30 mg/kg while splenic levels of the cytokines IFNγ and TNFα were decreased at ≥ 3 mg/kg. Uranyl nitrate hexahydrate decreased the cytotoxicity of splenic natural killer cells at all dose levels. The most pronounced changes were seen at the highest dose. No effect was seen on behaviour, body weight, organ weight or blood-biochemical parameters used to assess hepatic and renal functions. Uranium accumulated in the kidney, sternum, spleen and thymus and was correlated with dose. A LOAEL level of 3 mg/kg of feed (equivalent to 0.4 mg uranium/kg bw per day) based on minor changes to the immune system (Hao et al., 2013a).
A similar study was conducted on 3 week-old Sprague-Dawley rats given feed containing 0, 1.3, 13 or 130 mg uranium/kg (equivalent to 0, 0.065, 0.65 or 6.5 mg uranium/kg bw per day) for 4 months. A significant, dose-dependent increase in uranium content was seen in the kidney, liver and spleen at all treated doses. Effects on immune function included delayed hypersensitivity induction at all doses and decreased lymphocyte count at 130 mg uranium/kg only. Histopathological changes were seen in the bone marrow (hyperplasia) and the spleen (decreased splenic white pulp, decreased number/volume of splenic corpuscles) and were most significant in the high dose group (Hao et al., 2013b).
9.2.2.4 Bone
Bones have a high affinity for uranium and are another target organ of uranium toxicity. Uranium exposure may interfere with normal bone formation functions (Arzuaga et al., 2015).
Male rats postnatally exposed for 9 months to drinking water containing 40 mg/L of uranyl nitrate had altered bone parameters (decreased cortical bone diameter, increased osteoid thickness, decreased mRNA expression of genes for normal bone structure and function) and altered renal function (significant decreased plasma creatinine, although within normal range for rats) while no effect was seen in rats when exposure began as adults (Wade-Gueye et al., 2012). Domingo et al. (1989a) found doses of ≥14 mg uranium/kg bw per day caused bipartite sternebrae, and developmental variations such as reduced ossification and unossified skeletal variations in offspring of 20 pregnant Swiss mice gavaged with 0, 5, 10, 25 or 50 mg uranyl acetate dihydrate/kg bw per day (equivalent to 0, 2.8, 5.6, 14 and 28 mg uranium/kg bw per day) on days 6–15 of gestation (see Section 9.2.5).
Adverse bone growth and formation were also seen in studies using other routes of exposure. Male Wistar rats (11/group) given a single i.p. injection of 2 mg uranyl nitrate/kg bw had altered architecture of the metaphyseal zone of the tibiae, shorter and wider trabeculae and less frequent osteoblastic areas. Bone formation surfaces were also significantly reduced (Guglielmotti et al., 1984). In another study, depressed bone formation (reduced bone growth in both tibiae and mandibles) was seen in rats (strain unspecified) given a single i.p. injection of 2 mg uranyl nitrate/kg bw or 30 dermal applications of 2.88 or 5.76 g U308 yellowcake/kg bw per day in petrolatum. High mortality was seen in all treatments (50%, 30% and 60% deaths, respectively by day 30) (Ubios et al., 1994-1995). Decreased bone formation and inhibition of endochondrial ossification in the tibia (shorter, and thinner, alterations of epiphyseal zone, less active osteoblasts) and mandible (marked by depletion of active osteoblasts and increased number of bone-lining cells on trabecular surface) were reported in rats subcutaneous implanted with insoluble uranium dioxide powder (0.125 g/kg bw) for 30 days (Diaz et al., 2002).
Despite a significant decrease in active vitamin D, in vitamin D receptor expression and mRNA levels of vitamin D target genes involved in renal calcium transport in rats given drinking water containing 40 mg/L of depleted uranium for 9 months, no effect in calcium or phosphate levels (i.e., mineral homoeostasis – important in bone formation) was observed (Tissandie et al., 2007). The activity and expression of CYP enzymes involved in vitamin D metabolism (crucial in the regulation of calcium-phosphate homeostasis) were altered in 10 week old Sprague-Dawley male rats given a single gavage dose of depleted uranium (204 mg uranyl nitrate/kg) (Tissandie et al., 2006). Decreased total bone mineral density in the proximal metaphysis of the tibia was observed at the highest dose in male Wistar rats given a single intramuscular dose of 0.2, 1.0 and 2.0 mg/kg bw mg depleted uranyl nitrate/kg bw and then sacrificed at 28 d. Bone biochemical markers were statistically significantly increased at all doses for osteocalcin, at 0.2 mg/kg bw for tartrate-resistant acid phosphatase and at 2.0 mg/kg bw for pyearidinodine and parathyroid hormone (Fukuda et al., 2006).
A single oral dose of 90 mg/kg bw of uranyl nitrate was enough to delay tooth eruption and tooth formation (indicators of bone formation/remodeling) in suckling Wistar rats exposed at postnatal day 1 or 7 during alveolar bone development when assessed 1 week post-exposure (Pujadas Biji et al., 2003). A follow-up study (Pujadas Biji and Ubios, 2007) showed that the initial impairment in tooth eruption and formation did not persist at 27 days post-exposure and that catch-up growth had occurred indicating reversibility. Tooth eruption was unaffected in Swiss mice pups whose mothers were dosed with 0.05, 0.5, 5 or 50 mg uranyl acetate dihydrate/kg per day starting at gestation day 13 (Domingo et al., 1989b; see Section 9.2.5). Decreased bone formation and increased bone resorption and resting bone in the periodontal bone of rats were seen following i.p. injections of 0.8 or 2 mg uranyl nitrate/kg bw (Ubios et al., 1991). Similarly, a significant decrease in bone formation (alveolar bone volume, total bone formation and volume density of bone) was observed in male Wistar rats (11/group) given a single i.p. injection of 2 mg uranyl nitrate/kg bw following extraction of 3 mandibular molars (Guglielmotti et al., 1984, 1985).
In vitro studies show that uranium interacts with proteins and genes involved in bone development, and also affect crystallinity and mineralization. The bovine serum protein fetuin-A (homologue of human α2-HS-glycoprotein) was found to have a high affinity for hexavalent uranium (U(VI) (the species of uranium present in aqueous solutions including blood) binding 3 U(VI) per protein and modifying the secondary structure of the protein, and possibly disrupting bone mineralization and bone metabolism regulation (Basset et al., 2013). Phosphorylated osteopontin (OPN), which is involved in bone mineralization, forms stable complexes with uranyl ions (9 equivalents of uranium/mole of protein) inducing conformational changes in the protein (Qi et al., 2014). Using DNA microarrays, Prat et al. (2010) showed that uranyl ions affected the transcriptional levels of SPP1 (secreted phosphoprotein 1 - a gene that codes for OPN) in human kidney cell lines thereby impacting the excretion of OPN in a time- and dose-dependent way. Using synthetic biomimetic apatites to model bone in vitro, Chatelain et al. (2015) found that uranium induced a loss of crystallinity and lowered the mineralization rate. An in vitro study using osteoblast-like ROS 17/2.8 rat-derived cells found uranium accumulated in cells and exerted cytotoxic effects after reaching a concentration threshold (≥75 uM) that varied with speciation of uranium in solution although alkaline phosphatase activity was not affected. In this study, two markers of bone formation and mineralization (bone sialoprotein and osteocalcin) were activated at low, non-toxic doses but inhibited at toxic doses (Milgram et al., 2008).
9.2.3 Long-term exposure and carcinogenicity
Earlier, lifetime feeding studies using diets containing up to 20% uranium reported decreased longevity in laboratory animals associated with exposure (Maynard and Hodge, 1949). A no-observed-effect-level (NOEL) (for the longevity) was reported to be 81 mg uranium/kg bw per day for rats exposed to uranyl fluoride. For other uranium compounds, NOELs of 1,130 mg uranium/kg bw per day (as uranyl nitrate), 1,390 mg uranium/kg bw per day (as uranium tetrafluoride) and 1,630 mg uranium/kg bw per day (as uranium dioxide) were reported for decreased longevity. Most deaths were associated with chemically-induced renal damage. Renal effects in rats included tubular necrosis, tubular lesion, and mild tubular degeneration following from chronic oral exposure to uranium. Hematological effects included anemia, and increased white blood cell count (Maynard and Hodge, 1949; Maynard et al., 1953). Other chronic effects included decreased body weight gain in rats. No histological effects were found in the spleen, lymph nodes, or bone marrow of various animals orally exposed (Maynard and Hodge, 1949; Tannenbaum and Silverstone, 1951; Maynard et al., 1953). The lowest NOEL value reported is 24 mg/kg bw per day for chronic renal effects in rats orally exposed to uranyl nitrate hexahydrate for one year (Maynard et al., 1953). The lowest reported NOEL and lowest-observed-effect-level (LOEL) values for haematological and renal effects were 19 and 39 mg/kg bw per day, respectively, in rats exposed to uranyl nitrate hexahydrate for two years (Maynard et al., 1953).
There are limited data on the carcinogenicity of uranium compounds in animals. Chronic oral exposure studies in rats and dogs have not reported neoplasms following ingestion of several uranium compounds at doses up to 12,341 mg uranium/kg per day for rats and 8,815 mg uranium/kg per day for dogs (Maynard and Hodge, 1949; Maynard et al., 1953).
9.2.4 Genotoxicity
Uranium's potential to induce genotoxicity has been shown in vivo following oral ingestion and in in vitro studies.
Weanling Wistar rats (50/sex/dose) were fed diets containing 0, 4, 40 mg uranium/kg bw per day as depleted uranyl nitrate for 4 months and then mated (F1). Offspring were exposed to the same doses as their parents for 4 months. The Comet assay and the bone marrow micronucleus test detected dose-dependent DNA damage that was more severe in the F1 groups. Changes in the histopathology of the testicles and the ovaries were also noted and were more pronounced in the F1 groups (Hao et al., 2009). A significant increase in bone marrow mutation frequency was also seen in the offspring of transgenic male mice given 50 mg uranium/L (equivalent to 1 mg per day) as depleted uranyl nitrate in drinking water for 2 months (Miller et al., 2010).
Positive results, including dose-dependent increases in uranium-DNA adduct formation and DNA damage, have been shown in in vitro studies including the chromosomal aberration assay, the HPRT assay, the micronucleus test, the sister chromatid exchange assay, the Comet assay and the cytokinesis-block-micronucleus centromere (CBMN) assay paired with the fluorescence in situ hybridization (FISH) assay (Lin et al., 1993; Stearns et al., 2005; Thiébault et al., 2007; Darolles et al., 2010). Negative findings for the Comet assay (Stearns et al., 2005) have also been observed.
9.2.5 Reproductive and developmental toxicity
Reproductive and developmental effects seen in animals following ingestion of soluble uranium compounds included: impaired female and male fertility, alterations in parental reproductive structures, fetotoxicity, and increased developmental variations. Effects on female fertility included oocyte damage (Feugier et al., 2008; Kundt et al., 2009), delayed folliculogenesis (Arnault et al., 2008), changes in the number of follicles, decreased pregnancy rate (Llobet et al., 1991; Radulescu et al., 2009) and number of pups per litter (Maynard and Hodge, 1949) with the lowest available NOAEL value being 10 mg uranyl nitrate hexahydrate/L (1.9 mg uranium/kg bw per day) based on oocyte quality (Feugier et al., 2008). A LOAEL of 5.6 mg uranium/kg bw per day for male fertility was available for Swiss mice, based on a decreased pregnancy rate in untreated females (Llobet et al., 1991). Maternal and fetotoxic effects showed a LOAEL of 2.8 mg uranium/kg bw per day in treated female Swiss mice (Domingo et al., 1989a).
Delayed folliculaogenesis was seen in two separate series of studies on ovarian function (Raymond-Whish et al., 2007; Arnault et al., 2008). Arnault et al. (2008) reported that uranium delayed folliculogenesis in vivo in mice at ≥5 mg uranyl nitrate/L (≥1.25 mg uranium/kg bw per day) and oocyte meiosis in vitro at 1 mM of uranyl acetate. In the first experiment, female mice (n = 5-10) were exposed for 15 weeks to drinking water containing 0, 5, 50 or 400 mg/L of uranyl nitrate (equivalent to 1.25, 12.5, 100 mg uranium/kg bw per day) and then either euthanized or mated (Experiment 2) with untreated males. Dams (Experiment 2) and their female pups (Experiment 3) were then followed for 3 months. Overall, treatment had no effect on body weight, general health, behaviour or kidney weights. Uranium did not accumulate in the ovaries. After 15 weeks of exposure, unmated females had fewer large antral follicles at all doses when compared to controls. These effects were also noted in the female pups. In experiment 2, increased secondary and early prenatal follicles were noted in all treated dams. A LOEL of 5 mg uranyl nitrate/L (1.25 mg uranium/kg bw per day) could be estimated. In the in vitro evaluation, mouse oocyte maturation was slowed down when cultured in the presence of 1 mM uranyl acetate (equivalent to 424 mg/L of uranyl nitrate).
A similar decrease in large primary follicles was seen at 0.5 mg uranyl nitrate hexahydrate/L (0.04 mg uranium/kg bw per day) only in immature B6C3F1mice (n=9-10/group) dosed with 0.5, 2.5, 12.5 and 60.0 mg uranyl nitrate hexahydrate /L (0, 0.04, 0.18, 0.89, 4.44 mg uranium/kg bw per day) for 30 days. Kidney weights were decreased in the mid (0.18 mg uranium/kg bw per day) and high (4.44 mg uranium/kg bw per day) dose groups. In a second experiment to assess effects of in utero exposure, B6C3F1 dams treated with 0.5, 0.25, 12.5 or 60 µg uranyl nitrate hexahydrate /L (0, 0.00004, 0.00018, 0.00089, 0.00444 mg uranium/kg bw per day) for 30 days prior to mating and then throughout gestation had a significant dose-dependent decrease in small primary follicles at ≥2.5 µg uranyl nitrate hexahydrate /L (0.00018 mg uranium/kg bw per day) while their female offspring showed a statistically significant decrease in primordial follicle numbers at 0.5 and 2.5 µg uranyl nitrate hexahydrate /L (0.00004 and 0.00444 mg uranium/kg bw per day) only. No gross anomalies were noted in any major organ examined. Ovariectomized C57B1/6J mice were also exposed to uranyl nitrate hexahydrate and exhibited changes in uterine morphology and histology, including significantly increased uterine weight. Overall, the findings suggest an estrogenic response to uranium (Raymond-Whish et al., 2007).
Fertility effects have been seen in early studies in which male and female rats were fed diets containing 2% uranyl nitrate hexahydrate for seven months, followed by normal diets for five months. Treated rats produced fewer litters, and fewer pups per litter (Maynard and Hodge, 1949). In follow-up studies, rats fed diets containing 2% uranyl nitrate hexahydrate for a single 24 hour period after weaning also produced fewer litters with fewer pups per litter than control rats, with no signs of maternal toxicity (Maynard et al., 1953). In a more recent study, no effects were observed in testicular function or spermatogenesis in male Swiss mice exposed for 64 days to uranyl acetate dihydrate in drinking water at doses of 0, 10, 20, 40 or 80 mg/kg bw/day (equivalent to 0, 5.6, 11.2, 22.4 and 44.8 mg uranium/kg bw per day) prior to mating with untreated females for 4 days (Llobet et al., 1991). Interstitial alterations and vacuolization of Leydig cells were observed at the highest dose while decreases in total epididymis weight and epididymis weight to body weight were seen at ≥11.2 mg uranium/kg bw per day. Pregnancy rate was significantly decreased at ≥5.6 mg uranium/kg bw per day although no differences in the number of live and dead foetuses were seen when compared with females mated with control males. A LOAEL of 5.6 mg uranium/kg bw per day for male fertility, based on decreased pregnancy rate, was determined (Llobet et al., 1991).
The combined action of stress and uranium was evaluated on a variety of outcomes including male reproduction (Linares et al., 2005). In this study, adult male rats (n=8) were exposed to 0, 10, 20 or 40 mg uranyl acetate dihydrate/kg bw/day (0, 5.6 11.2, 22.4 mg uranium/kg bw per day) in drinking water for 3 months. Half of the animals were exposed to 2 hours per day of restraint stress. Although histopathology revealed little effect, fertility reductions at 11.2 mg uranium/kg bw per day (non-dose dependent) only and spermatid numbers per testis at all doses were noted. Restraint did not enhance the uranium-induced effects. In subsequent analyses of the data, Linares et al. (2006 and 2007) reported evidence of pro-oxidant activity of uranium in the testis, kidney and brain of rats. In the testes, glutathione reductase and glutathione were decreased while superoxide dismutase was increased. Evidence of pro-oxidant activity in the kidney and brain included increased thiobarbituric acid-reactive substances and oxidized glutathione and superoxide dismutase activity.
Decreased fertility was also seen in uranium-treated pregnant Sprague-Dawley rats gavaged with either 0.830 or 1 mg uranyl acetate dehydrate/kg/d from gestation day 5 to 16. Decreased maternal body weight gain and terminal body weight was seen in treated rats and was accompanied by a decrease in food intake. A significant decrease in fertility (expressed as decreased foetal viability and litter size and increased spontaneous abortions) was also noted. No gross foetal external abnormalities or histological effects in bone were noted, although pup size and weight were both significantly decreased at 0.830 mg uranyl acetate dehydrate/kg/d (Radulescu et al., 2009).
Fetotoxic and developmental effects were seen in several studies. Domingo et al. (1989a) evaluated the developmental toxicity of uranium by treating groups of 20 pregnant Swiss mice by gavage to doses of 0, 5, 10, 25 or 50 mg uranyl acetate dihydrate/kg bw/day (equivalent to 0, 2.8, 5.6, 14 and 28 mg uranium/kg bw per day) on days 6–15 of gestation; the animals were sacrificed on day 18. Dose-dependent maternal toxicity was seen starting at the lowest dose (2.8 mg uranium/kg bw per day). Exposure-related fetotoxicity consisting of reduced foetal body weights and an increase in total external defects was seen starting at 2.8 mg uranium/kg bw per day while reduced foetal body length, and increased incidence of both stunted fetuses per litter and developmental variations were seen starting at 5.6 mg uranium/kg. At doses of ≥14 mg uranium/kg bw per day, specific malformations included cleft palate and bipartite sternebrae, and developmental variations such as reduced ossification and unossified skeletal variations were observed. Delayed development of renal papillae was observed at 2.8 and 14 mg uranium/kg bw per day but was not statistically significant. There was no evidence of embryolethality at any dose. A LOAEL of 2.8 mg uranium/kg bw per day could be determined based on both the maternal and fetotoxic effects in mice (Domingo et al., 1989a).
A second study by Domingo et al. (1998b) evaluated the effect of uranium on late foetal development, parturition, lactation and postnatal viability. Groups of 20 female mice were treated by gavage from day 13 of pregnancy until day 21 of lactation to doses of 0, 0.05, 0.5, 5 or 50 mg uranyl acetate dihydrate/kg bw per day (equivalent to 0, 0.028, 0.28, 2.8 and 28 mg uranium/kg bw per day). Maternal deaths (2/20 and 3/20 at the two highest doses, respectively) were attributed to the treatment; however, maternal toxicity was not evident from changes in body weight or food consumption, although relative liver weight was significantly reduced in all treatment groups. No effect on incisor eruption (indicative of bone formation remodeling) was seen at any dose. Decreases in pup viability, as indicated by significant decreases in litter size on day 21 of lactation, and significant decreases in the viability and lactation indexes were observed in the highest dose group. Based on developmental effects in pups, the NOAEL is 2.8 mg uranium/kg bw per day.
A significant decrease in fetal body weight (at 1 and 2 mg uranyl acetate dihydrate/kg bw per day) and a significant increase in total skeletal effects (decreased ossification or no ossification in a number of bones in all doses) were observed in fetuses of Swiss mice dams (25/dose) given subcutaneous injections of 0.5, 1, 2 mg uranyl acetate dihydrate/kg bw per day from gestation day 6 to 15 (period of organogenesis). High maternal toxicity, including death (30% mortality in high dose group) was also observed which may have contributed to the fetotoxicity observed. Other maternal and embryo/fetotox effects were also noted (Bosque et al., 1993).
Paternain et al. (1989) studied the effects of uranium on reproduction, gestation and postnatal survival. Swiss mice (25/sex/group) were gavaged with 0, 5, 10 or 25 mg uranyl acetate dihydrate/kg bw per day (equivalent to 0, 2.8, 5.6 or 14.0 mg uranium/kg bw per day). Males were dosed for 60 days prior to mating while females were dosed starting at 14 days prior to mating and continuing until day 21 of lactation, unless sacrificed at day 13 of gestation. Significant reproductive effects were seen at 14 mg uranium/kg bw per day and consisted of an increased number of total and late resorptions, increased number of dead foetuses and a decrease in both the number of live foetuses and in the viability indices. There was a significant decrease in the number of litters over time (measured at PND 0, 4 and 21) starting at 5.6 mg uranium/kg bw per day). Pup growth was supressed at 2.8 mg uranium/kg bw per day for body weight and 5.6 mg uranium/kg bw per day) for body length. The authors gave a NOEL of 5 mg uranyl acetate dihydrate/kg bw/day, equivalent to 2.8 mg uranium/kg bw per day.
Eight groups (2 groups/dose) of male Sprague-Dawley rats (16/group) were given drinking water containing 0, 10, 20 or 40 mg uranyl acetate dihydrate/kg bw per day (0, 5.3, 11.2, 22.4 mg uranium/kg bw per day) for 3 months. One group per dose was exposed to 2 hrs of restraint stress per day. At the end of 3 months, males were mated with untreated females. At 20 mg/kg bw per day only pregnancy rate was significantly decreased (not dose-dependent). No significant effect was seen on the physical maturation of offspring (as measured by number of implants/litter, the number of dead/resorbed foetuses, viability index, lactation index or the number of days to pinna detachment or eye opening, body weight), neuromotor maturation (as measured by surface righting and forelimb grip strength) or behaviour (as measured by passive avoidance acquisition and mean distance traveled on trial probe). Slight differences were seen in the negative geotaxis test in female offspring only. These differences were transient and not dose-dependent. Isolated differences were also seen in the water maze test but these were independent of dose used. The NOAEL was 10 mg/kg bw per day of uranyl acetate dehydrate (calculated as 5.6 mg uranium/kg bw per day) (Albina et al., 2005).
A single oral dose of 90 mg/kg bw of uranyl nitrate was enough to delay tooth eruption and tooth formation in suckling Wistar rats exposed at postnatal day 1 or 7 during alveolar bone development when assessed 1 week post-exposure. Histopathological changes (hyaline casts and cell vacuolization were also seen in the kidneys (Pujadas Biji et al., 2003). A follow-up study (Pujadas Biji and Ubios, 2007) showed that the initial impairment in tooth eruption and formation did not persist at 27 days post-exposure and that catch-up growth had occurred.
9.3 Mode of action
Uranium appears to cause nephrotoxicity through its interaction with the proximal tubules. In humans, chronic exposure to uranium via drinking water was associated with elevated biomarkers of proximal tubule damage (i.e., increased urinary excretion of glucose, ions and low molecular weight proteins), and to a lesser extent, glomerular dysfunction (i.e., albuminuria) (Kurttio et al., 2002, 2006a; Mao et al., 1995; Zamora et al., 1998). In animal studies, histological analysis has indicated damage to both the glomerulus and tubules (Ortega et al., 1989; Gilman et al., 1998a, 1998b, 1998c; Donnadieu-Clarez et al., 2007; Berradi et al., 2008).
The proposed mode of action for nephrotoxicity relating to uranium exposure appears to involve three steps: uranyl ion forms complexes with bicarbonate ions and proteins in the blood, these complexes are taken up by the kidneys leading to impaired kidney function. Once in the blood (Adams and Spoor, 1974; Osman et al., 2015), uranium is predominantly present as the uranyl ion (Durbin and Wrenn, 1976; Keith et al., 2007) which mostly complexes with bicarbonate anions and with plasma proteins such as transferrin and albumin (Butterworth, 1955; Ballou et al., 1986; Diamond et al., 1989; Kocher, 1989; Leggett, 1989; National Research Council, 1999). The bicarbonate complex is readily filtered through the glomerulus and enters the proximal tubular fluid where, under the reduced pH of the tubules, it dissociates. As bicarbonate, citrate, and water are reabsorbed in the proximal tubules, the concentration of uranyl ions in the tubular fluid increases (Durbin and Wrenn, 1976; National Research Council, 1988; Beckett et al., 2007; ATSDR, 2013). The freed uranyl ions can then complex with phosphate ligands on the luminal surface of the tubule cells, impairing kidney function (Beckett et al., 2007). Uranium can also specifically bind to a small number of proteins in the kidney involved in iron homeostasis and gene expression (Frelon et al., 2009). The undissociated bicarbonate complex is not absorbed and passes directly into the urine. As with other weak acids, the pH in the lumen and the presence of bicarbonate influence the rate of uranium elimination in the urine. Little dissociation of the bicarbonate complex occurs when alkalinity is increased, resulting in increased urinary excretion of uranium while acidification of the urine results in almost complete dissociation of the complex, leading to a large renal uptake of bicarbonate and a small excretion of uranium (Beckett et al., 2007; McDiarmid et al., 2012).
In general, the stage of renal development, especially the maturity of the renal tubular transport process, impacts the severity of the toxic effects seen in the kidneys. Increased tubular transport generally means increased tubular toxicity. Human renal development involves two basic processes – morphological formation (completed in utero) and acquisition of function (begins with earliest formation of foetal nephrons and accelerates after birth to reach adult levels). In humans, nephrogenesis occurs between the 6th and the 36th week of gestation, after which time the anatomic formation of the kidney is complete with a full complement of nephrons. The primary functions of the kidney (glomerular filtration, tubular secretion and tubular reabsorption) are dependent on renal blood and renal plasma flow which increase with age as a result of an increase in cardiac output and a reduction in peripheral vascular resistance (Fernandez et al., 2011). At birth, these primary kidney functions are all decreased (Lee, 2009; Fernandez et al., 2011). Newborns also have a limited ability to concentrate urine and have lower urinary pH values (Lee, 2009). Accelerated kidney growth and physiological changes occur postnatally, with kidney function reaching adult levels at 1 to 2 years of age. Prior to this, the low renal blood flow and decreased tubular transport seen in infants may serve to protect the kidneys from toxic effects (Solhaug, 2004). As nephron formation continues after birth in animals, the results of studies on renal function in rodents and other experimental models need to be interpreted carefully (Solhaug, 2004).
Other proposed mechanisms include the interference by uranium of ATP utilization and mitochondrial oxidative phosphorylation in the renal proximal tubule leading to disruption of active transport in the cells of the renal proximal tubules and associated structures (Brady et al., 1989; SCAHT, 2015). Formation of reactive oxygen species, possibly related to disruption of iron homeostasis (Donnadieu-Clarez et al., 2007) and leading to altered gene, protein and enzyme expression in the kidneys, testes and brain has also been proposed (Taulan, et al., 2004; Linares et al., 2006, 2007; Donnadieu-Clarez et al., 2007).
Uranium may also cause bone effects by decreasing cell proliferation in areas of endochondral ossification, altering the cytoplasm and nuclei of active and inactive osteoblasts, decreasing the number of active osteoblasts, and inhibiting the expression of genes associated with normal bone development and mineralization (Wade-Gueye et al., 2012; Arzuaga et al., 2015). It has also been reported that uranium can alter mineral homeostasis by binding to osteoponin, a protein expressed in mineralized tissues (Arzuaga et al., 2015).
10.0 Classification and assessment
With respect to chemical toxicity, there is insufficient evidence to conclude that oral exposure to natural uranium will cause cancer in humans or animals (National Research Council, 1988; U.S. EPA, 1989; EFSA, 2009; ATSDR, 2013). No major health agency has provided a cancer slope factor for uranium. Natural uranium has not been classified as a carcinogen by the National Toxicology Program (NTP), the International Agency for Research on Cancer (IARC) or by the U.S. EPA. The National Research Council (1988) looked at the carcinogenic effects of internally deposited uranium in an attempt to provide a quantitative risk estimate on cancer induction. It determined that the most likely cancer resulting from uranium exposure, specifically from highly enriched uranium, would be bone sarcomas. No epidemiological studies have shown an increase in such cancers and the National Research Council (1988) concluded that natural uranium may have no measurable carcinogenic effects.
Only a few exploratory epidemiological studies relating to drinking water as a route of exposure were available and their results are equivocal (see Sec. 9.1.3). Despite genotoxicity being reported in in vitro studies (see Sec. 9.2.4) and in a limited number of in vivo studies (Hao et al., 2009; Miller et al., 2010), no specific cancer studies in animals using oral exposure were located. In the few long-term oral studies available (Maynard and Hodge, 1949; Maynard et al., 1953; Donnadieu-Clarez et al., 2007; Gueguen et al., 2014) no neoplasms were noted in either dogs or rats following ingestion of several uranium compounds. Therefore, the available human and animal data are inadequate to perform a quantitative cancer risk assessment.
10.1 Non-cancer risk assessment
In epidemiological studies, only kidney effects have been consistently found in humans following natural uranium exposure while the main target organ for animals is the kidneys (Maynard and Hodge, 1949; Tannenbaum and Silverstone, 1951; Goel et al., 1979; Ortega et al., 1989; Mao et al., 1995; Gilman et al., 1998a, 1998b; Zamora et al., 1998; Kurttio et al., 2002, 2006a). The lowest available point of departure found in animal studies is based on kidney effects and is a LOAEL of 0.06 mg/kg bw per day (Gilman et al., 1998a). Uranium accumulates mainly in bone and to a lesser extent in the kidneys, with toxic effects seen in both organs. The evidence for bone effects suggests a hazard associated with uranium exposure but the available studies are inadequate for risk assessment due to their study designs (only single and mostly high or lethal doses used), routes of exposure (mostly injection studies) and inconsistent results (genes/protein expression altered but overall process unaffected). Overall, the literature shows uranium does have an effect on bone development and maintenance but studies are insufficient to provide a NOAEL/LOAEL with the exception of Domingo et al. (1989a) which showed fetal skeletal effects at ≥14 mg uranium/kg bw per day in the presence of high maternal toxicity. This LOAEL for bone effects is much higher than the lowest available LOAEL of 0.06 mg/kg bw per day for kidney effects from Gilman et al. (1998a).
As such, the kidney is likely the main target organ of uranium toxicity and the best-characterized based on current available literature. There is no indication that infants or children would be more sensitive to kidney effects. In fact limited animal studies in adult rats and mice suggest adults may be more sensitive to kidney effects than weanling animals to uranium (Maynard and Hodge, 1949; Tannenbaum and Silverstone, 1951; Homma-Takeda et al., 2013).
In epidemiological studies, chronic exposure to uranium via drinking water has been associated with elevated biomarkers of proximal tubule damage (i.e., increased urinary excretion of glucose, ions and low molecular weight proteins), and to a lesser extent, glomerular dysfunction (i.e., albuminuria) (Mao et al., 1995; Zamora et al., 1998; Kurttio et al., 2002, 2006a) although biomarker values were often inconsistent among studies and within normal clinical ranges. Additionally, most of the study populations were quite small (i.e., n=50-300) and had poor exposure estimates that relied on a minimal number of urine samples and on self-reported food and drink consumption, factors which are not representative of long-term uranium exposure and can induce bias into the results. Other studies showed no evidence of effects on renal proximal tubule function and no clear signs of renal damage (Seldén et al., 2009), although a weak association was seen between cumulative uranium intake and glucose levels in the urine as well as in increased blood pressure (Kurttio et al., 2006a). Little information is available relating to exposure of children. The one available study showed elevated β2-microglobulin in a 3 year old child but not in her 4 older siblings (Magdo et al., 2007). A no-effect concentration could not be derived from the human studies available to date. Overall, the epidemiological evidence does not provide a reliable dose-response characterization as noted by ATSDR (2013). However, the mild kidney effects seen in the epidemiological studies, most notably in the Kurttio et al. (2002) study, are consistent with the kidney effects found in animals.
Renal damage was reported in a number of studies in several species (rats, dogs, rabbits) of animals (Maynard and Hodge, 1949; Tannenbaum and Silverstone, 1951; Ortega et al., 1989; Gilman et al., 1998a and1998b; Craft et al., 2004; Brugge and Buchner, 2011; ATSDR, 2013). Reported kidney effects varied in intensity, ranging from microscopic lesions to necrosis and included changes in blood chemistry, urinary markers and gene expression relating to kidney function, red blood cell counts, and iron metabolism, although some studies found no marked kidney effects even at doses of 40 mg uranium /kg bw per day given for up to 9 months (Maynard and Hodge, 1949; Tannenbaum and Silverstone, 1951; Goel et al., 1979; Ortega et al., 1989; Gilman et al., 1998a, 1998b; Taulan, 2004; Donnadieu-Claraz et al., 2007; Berradi et al., 2008; Rouas et al., 2011; Grison et al., 2013; Poisson et al., 2014a and 2014b). Other studies showed that once exposure ceased, uranium was efficiently removed from the body and the observed kidney effects were reversible (Maynard and Hodge, 1949; Morrow et al., 1982; Bentley et al., 1985; Diamond et al., 1989; Leggett, 1989; Orloff et al., 2004; Tessier et al., 2012).
Rats were considered the most sensitive species to uranium toxicity following chronic exposure (Vicente-Vicente et al., 2010). LOAEL values for kidney effects ranged from 0.06 to 5.6 mg uranium/kg bw per day in rats and often represented the lowest dose tested (Ortega et al., 1989; Gilman et al., 1998a, 1998b, 1998c; Linares et al., 2006). The most severe kidney effects were reported in a 3 month study by Gilman et al., (1998a) with a LOAEL of 0.06 mg uranium/kg bw per day for male rats. More recent studies using methodologies similar to Gilman et al. (1998a) but with an extended dosing period of up to 9 months did not report significant changes in kidney pathology and determined a NOAEL of 5.4 mg/kg bw per day (the highest dose tested) (Dublineau et al., 2014; Gueguen et al., 2014; Poisson et al., 2014a, 2014b). Studies showed that although the amount of uranium absorbed from the GI tract was lower in rats than in humans (see Sec. 8.1), the proportion of absorbed uranium distributed and retained in the skeleton and kidneys was similar in rats and humans (see Sec. 8.2) making the rat an appropriate surrogate for uranium toxicity in humans.
As a conservative approach, the Gilman et al. (1998a) study was selected as the point of departure for this risk assessment as it was the lowest available LOAEL for kidney effects. As the kidney data did not show a strong correlation between dose and response, Benchmark Dose Modeling could not be used (ATSDR, 2013). Gilman et al. (1998a) reported statistically significant changes in the kidney for all doses tested and established a LOAEL of 0.96 mg uranyl nitrate hexahydrate/L (corresponding to 0.06 mg uranium/kg bw per day for males and 0.09 mg uranium/kg bw per day for females). Despite study shortcomings, such as the lack of an identified NOAEL, no reported clinical chemistry, and a poor dose-response relationship, the histopathological changes seen in the kidney, although more severe, were consistent with other studies and were supported by a plausible mode of action (Maynard and Hodge, 1949; Tannenbaum and Silverstone, 1951; Goel et al., 1979; Ortega et al., 1989; Gilman et al., 1998b, 1998c). Although the Gilman et al. (1998b) rabbit study had a slightly lower LOAEL of 0.05 mg uranium/kg bw per day (0.96 mg uranyl nitrate hexahydrate/L) for renal toxicity, it was not used to estimate a tolerable daily intake (TDI) since male rabbits were infected with Pasturella multocida (an infection that may develop into septicemia leading to acute death in the absence of any clinical signs; Aiello et al., 2012), potentially confounding the results. Other possible points of departure based on kidney effects (a NOAEL of 1 mg/kg bw per day (Ortega et al., 1989) and a LOAEL of 5.6 mg uranium/kg bw per day (Linares et al., 2006)) are 16 and 93 times higher than that based on Gilman et al. (1998a). Based on the above considerations, adding an uncertainty factor to account for using a LOAEL instead of a NOAEL was not considered necessary.
Changing the length of exposure had inconsistent results on histopathological effects in the kidney. Comparing 3 month and 9 month exposures in rats, Poisson et al. (2014b) did not observe differences in kidney histopathology related to exposure time or dose, while Hamid (2012) observed more pronounced renal histopathological findings in rats gavaged with 35 mg uranium /kg bw per day for 60 days compared to those gavaged for 30 days. Sprague-Dawley rats given 2.7 mg uranium/kg bw per day in drinking water for 6, 9, 12 or 18 months had significant changes in proximal tubular cells at 6 and 9 months of exposure but by 12 and 18 months, normalization of the epithelia could be seen although debris was still present in the tubule lumen (Donnadieu-Claraz et al., 2007). Berradi et al. (2008) noted an increase in the severity of tubule-interstitial lesions but not in their frequency in Sprague-Dawley rats given 2.4 mg uranium/kg bw per day in drinking water for 9 months. Studies dosing rats for up to 9 months did not report significant changes in kidney pathology and determined a NOAEL of 5.4 mg/kg bw per day or 90 times higher than the LOAEL of Gilman et al. (1998a) (Dublineau et al., 2014; Gueguen et al., 2014; Poisson et al., 2014a, 2014b). In addition, the use of a subchronic study for estimation of a TDI was adequately sensitive and did not require an additional uncertainty factor since the observed kidney effects were similar to the minimal effects seen in a number of longer-term studies and since human absorption values were found to be independent of exposure duration (Limson Zamora et al. 2002a, 2002b and 2003).
An uncertainty factor of 100 (´10 for interspecies variability, ´10 for intraspecies variability) was applied to the LOAEL.
A TDI of 0.0006 mg uranium/kg bw per day, therefore can be derived as follows:
TDI = 0.06 mg/kg bw per day ÷ 100
= 0.0006 mg/kg bw per day
where:
- 0.06 mg/kg bw per day is the LOAEL from the Gilman et al. (1998a) rat study, based on an increase in renal lesions in male rats; and
- 100 is the uncertainty factor (×10 for interspecies variability, ×10 for intraspecies variability).
Using the TDI derived above, a health-based value (HBV) for total natural uranium in drinking water can be calculated as follows:
HBV = 0.0006 mg/kg bw per day ×70 kg × 0.50 ÷ 1.5 L/d
= 0.014 mg/L
where:
- 0.0006 mg/kg bw per day is the TDI derived above;
- 70 kg is the average body weight of an adult (Health Canada, 1994);
- 0.5 is the allocation factor estimated for drinking water (see Sec. 5.0); and
- 1.5 L/day is the daily average volume of drinking water ingested by an adult (Health Canada, 1994).
10.2 International considerations
Other organizations have set guidelines or regulations pertaining to the concentration of uranium in drinking water. WHO (2011) provides a provisional guideline value of 30 µg/L based on an epidemiological study by Kurttio et al. (2006a). This value is considered to be provisional since there is uncertainty regarding the toxicological and epidemiological dataset and concern over the technical achievability of the guideline value (particularly in smaller communities). Frisbie et al. (2013, 2015) points out that the Kurttio et al. (2006a) study is actually a subset of a larger study (Kurttio et al., 2002) and that the “no effect” group is actually associated with increased systolic blood pressure and urinary glucose levels. The U.S. EPA (2009) established a regulatory maximum contaminant level (MCL) of 30 µg/L, Australia adopted a guideline of 17 µg/L (NHMRC, 2011) and California established a non-regulatory public health goal of 0.5 µg/L (OEHHA, 2001). Both the U.S. EPA MCL and the Australian guideline were established using the TDI based on the Gilman et al. (1998a) rat study. As of 2018, the European Union has not established a drinking water value for uranium.
11.0 Rationale
Uranium is widespread in nature; it exists in combination with other elements and has been identified in up to 150 different minerals. Uranium exists in several chemical oxidation states. The principal health effects associated with natural uranium are due to its chemical toxicity. Uranium can enter drinking water sources through weathering and leaching from natural deposits, fallout from volcanic eruptions, mill tailings releases, emissions from the nuclear industry and the combustion of coal and other fuels, as well as phosphate/nitrate fertilizer use.
Insufficient evidence exists to conclude that oral exposure to natural uranium will cause cancer in humans or animals. Despite genotoxicity being reported in in vitro and in vivo studies, no specific cancer studies in animals using oral exposure are available.
The kidney is generally considered as the major target for uranium toxicity in both humans and animals. Epidemiological studies provide some evidence of an association between chronic exposure to uranium via drinking water and elevated biomarkers of kidney effects, however, study weaknesses and a lack of reliable dose-response characterization prevent these studies from being used for risk assessment. Kidney damage has been reported in a number of animal species with rats appearing to be the most sensitive following chronic exposure. Given that the distribution and retention of absorbed uranium in the kidneys (and bone) in rats is similar to that in humans, data from rats is deemed as most appropriate for the basis of a MAC.
Uranium is naturally found in groundwater in many regions of the country. Exposure to uranium is primarily from natural sources and is related to local and regional geology. The calculation of the HBV is based on a conservative estimate of the effects of natural uranium on the kidney. However, based on its low absorption and limited evidence that effects on the kidney do not increase with increasing exposure, it was determined that the difference in health protection between the calculated HBV and the current MAC is not expected to be significant. In addition, there are anticipated challenges and operational cost impacts, particularly for private wells and in small communities, to meet the HBV of 0.014 mg/L (14 µg/L) when, based on the available science, no appreciable health benefits would be expected when compared to the current MAC. As such, the maximum acceptable concentration of 0.02 mg/L (20 µg/L) is maintained for total natural uranium in drinking water. As part of its ongoing guideline review process, Health Canada will continue to monitor new research in this area and recommend any change to the guideline that is deemed necessary.
12.0 References
Acquavella, J.F., Wiggs, L.D., Waxweiler, R.J., Macdonell, D.G., Tietjen, G.L. and Wilkinson, G.S. (1985). Mortality among workers at the Pantex weapons facility. Health Phys., 48(6): 735–746.
Adams, N. and Spoor, N.L. (1974). Kidney and bone retention functions in the human metabolism of uranium. Phys. Med. Biol., 19(4): 460–471.
Ahier, B.A. and Tracy, B.L. (1997). Evaluating the radiological impact of uranium emissions in Port Hope, Ontario—A comparison of monitoring and modelling results. J. Environ. Radioact., 34(2): 187–205.
Aiello, S.E., Moss, M.A. and Steigerwall, M.A. (eds.)(2012). The MERCK veterinary manual. 10th edition. The Merck Publishing Group, Online. Available at: www.merckvetmanual.com/mvm/index.html.
Akyil, S., M.A.A. Aslani, and M. Eral. (2003). Adsorption characteristics of uranium onto composite ion exchangers. J. Radioanal. Nucl. Chem., 256: 45–51.
Alberta Environment and Parks (2015). Personal communication with D. Reid, Drinking Water and Waste Water.
Albina, M.L., Bellés, M., Linares, V., Sánchez, D.J. and Domingo, J.L. (2005). Restraint stress does not enhance the uranium-induced developmental and behavioral effects in the offspring of uranium-exposed male rats. Toxicology, 215(1–2): 69–79.
Al-Jundi, J., Werner, E., Roth, P., Höllriegl, V., Wendler, I. and Schramel, P. (2004). Thorium and uranium contents in human urine: Influence of age and residential area. J. Environ. Radioact., 71(1): 61–70.
Anke, M., Seeber, O., Müller, R., Schäfer, U. and Zerull, J. (2009). Uranium transfer in the food chain from soil to plants, animals and man. Chemie Der Erde - Geochemistry, 69 (S2): 75–90.
Ankomah, A.B. (1991). Filtration-rate technique for determining zero point of charge of iron oxides. Clays Clay Miner., 39(1): 100–102.
APHA, AWWA and WPCF (1989). Standard methods for the examination of water and wastewater. Clesceri, L.S., Greenberg, A.E., Trussell, R.R. (eds.). 17th Edition. American Public Health Association, American Water Works Association, Water Pollution Control Federation, Washington, DC.
APHA, AWWA and WEF (1998). Standard methods for the examination of water and wastewater. Rice, E.W., Baird, R.B., Eaton, A.D. and Clesceri, L.S. (eds.). 20th edition. American Public Health Association, American Water Works Association, Water Environment Federation, Washington, DC.
APHA, AWWA and WEF (2005). Standard methods for the examination of water and wastewater. Eaton, A.D., Clesceri, L.S., Rice, E.W., Greenberg, A.E. (eds.). 21st edition. American Public Health Association, American Water Works Association, Water Environment Federation, Washington, DC.
APHA, AWWA and WEF (2017). Standard methods for the examination of water and wastewater. Baird, R.B., Eaton, A.D., Rice, E.W. (eds.). 23rd edition. American Public Health Association, American Water Works Association, Water Environment Federation, Washington, DC.
Arey, S,. Seaman, J. and Bertsch, P. (1999). Immobilization of uranium in contaminated sediments by hydroxyapatite addition. Environ. Sci. Technol., 33: 337–342.
Arnault, É., Doussau, M., Pesty, A., Gouget, B., Van der Meeren, A., Fouchet, P. and Lefèvre, B. (2008). Natural uranium disturbs mouse folliculogenesis in vivo and oocyte meiosis in vitro. Toxicology, 247(2–3): 80–87.
Artinger, R., Rabung, T., Kim, J., Sachs, S., Schmeide, L., Heise, K., Bernhard, G. and Nitsche, N. (2002). Humic colloid-borne migration of uranium in sand columns. J. Contaminant Hydrology, 58: 1– 12.
Arzuaga, X., Gehlhaus, M. and Strong, J. (2015). Modes of action associated with uranium induced adverse effects in bone function and development. Toxicol. Lett., 236: 123–130.
ASTM (1991a). ASTM D2907–91 Standard test methods for microquantities of uranium in water by fluorometry. ASTM International, West Conshohocken, Pennsylvania.
ASTM (1991b). ASTM D5174–91 Standard test method for trace uranium in water by pulsed-laser phosphorimetry. ASTM International, West Conshohocken, Pennsylvania.
ASTM (1997a). ASTM D2907–97 Standard test methods for microquantities of uranium in water by fluorometry (withdrawn 2003). ASTM International, West Conshohocken, Pennsylvania.
ASTM (1997b). ASTM D5174–91 Standard test method for trace uranium in water by pulsed-laser phosphorimetry. ASTM International, West Conshohocken, Pennsylvania.
ASTM (2002). ASTM D5174–02 Standard test method for trace uranium in water by pulsed-laser phosphorimetry. ASTM International, West Conshohocken, Pennsylvania.
ASTM (2003). ASTM D5673–03 Standard test method for elements in water by inductively coupled Plasma—Mass spectrometry. ASTM International, West Conshohocken, Pennsylvania.
ASTM (2005). ASTM D5673–05 Standard test method for elements in water by inductively coupled Plasma—Mass spectrometry. ASTM International, West Conshohocken, Pennsylvania.
ASTM (2007). ASTM D5174–07 Standard test method for trace uranium in water by pulsed-laser phosphorimetry. ASTM International, West Conshohocken, Pennsylvania.
ASTM (2010). ASTM D5673–10 Standard test method for elements in water by inductively coupled Plasma—Mass spectrometry. ASTM International, West Conshohocken, Pennsylvania.
ASTM (2016). ASTM D5673–05 Standard test method for elements in water by inductively coupled plasma—Mass spectrometry. ASTM International, West Conshohocken, Pennsylvania.
ATSDR (2013). Toxicological profile for uranium. Agency for Toxic Substances and Disease Registry, Public Health Service, U.S. Department of Health and Human Services, Atlanta, Georgia.
Austin, A.L., Ellender, M., Haines, J.W., Harrison, J.D. and Lord, B.I. (2000). Microdistribution and localized dosimetry of the α-emitting radionuclides 239Pu, 241Am and 233U in mouse femoral shaft. Int. J. Radiat. Biol., 76(1): 101–111.
Auvinen, A., Kurttio, P., Pekkanen, J., Pukkala, E., Ilus, T. and Salonen, L. (2002). Uranium and other natural radionuclides in drinking water and risk of leukemia: A case-cohort study in Finland. Cancer Causes & Control, 13(9): 825–829.
Auvinen, A., Salonen, L., Pekkanen, J., Pukkala, E., Ilus, T. and Kurttio, P. (2005). Radon and other natural radionuclides in drinking water and risk of stomach cancer: A case-cohort study in Finland. Int. J. Cancer, 114: 109–113.
Ayotte, J.D., J.M. Gronberg, and L.E. Apodaca. (2011). Trace Elements and radon in groundwater across the United States, 1992-2003. Scientific Investigations Report 2011–5059, U.S. Geological Survey, Reston, Virginia.
Aytas, S., S. Akyil, and M. Eral. (2004). Adsorption and thermodynamic behavior of uranium on zeolite. J. Radioanal. Nucl. Chem., 260: 119–125.
Bachman, J. (1984). Field and laboratory analysis of water from Columbia aquifer in Eastern Maryland. Groundwater,.22 (4):460-467.
Baeza, A., Fernandez, M., Herranz, M., Legarda, F., Miro, C., and Salas, A. (2006). Removing uranium from natural water. Air, water and soil pollution, 173: 57–60.
Ballou, J.E., Gies, R.A., Case, A.C., Haggard, D.L., Buschbom, R.L. and Ryan, J.L. (1986). Deposition and early disposition of inhaled 234UO2(NO3)2 and 232UO2(NO3)2 in the rat. Health Phys., 51(6): 755–771.
Bang, S. and Meng, X.. (2004). A review of arsenic interactions with anions and iron hydroxides. Environ. Eng. Res., 9(4): 184–192.
Basset, C., Averseng, O., Ferron, P.J., Richaud, N., Hagège, A., Pible, O. and Vidaud, C. (2013). Revision of the biodistribution of uranyl in serum: Is fetuin-A the major protein target? Chem. Res. Toxicol., 26: 645–653.
Beckett, W.S., Nordberg, G.F. and Clarkson, T.W. (2007). Chapter 3 – Routes of exposure, dose, and metabolism of metals. In: Handbook on the toxicology of metals (third edition). Nordberg, G.F., Fowler, B.A., Nordberg, M. and Friberg, L.T. (eds.). Academic Press, Burlington, Massachussetts, pp. 39–64.
Bellés, M., Albina, M.L., Linares, V., Gómez, M., Sánchez, D.J. and Domingo, J.L. (2005). Combined action of uranium and stress in the rat: I. Behavioral effects. Toxicol. Lett., 158(3): 176–185.
Bensoussan, H., Grandcolas, L., Dhieux-Lestaevel, B., Delissen, O., Vacher, C.-M., Dublineau, I., Voisin, P., Gourmelon, P., Taouis, M. and Lestaevel, P. (2009). Heavy metal uranium affects the brain cholinergic system in rats following sub-chronic and chronic exposure. Toxicology, 261: 59–67.
Bentley, K.W., Stockwell, D.R., Britt, K.A. and Kerr, C.B. (1985). Transient proteinuria and aminoaciduria in rodents following uranium intoxication. Bull. Envion. Contam. Toxicol., 34: 407–416.
Berglund, M., Lindberg, A.L., Rahman, M., Yunus, M., Grandér, M., Lönnerdal, B. and Vahter, M. (2011). Gender and age differences in mixed metal exposure and urinary excretion. Environ. Res., 111(8): 1271–1279.
Berlin, M. and Rudell, B. (1986). Uranium. In: Handbook on the toxicology of metals. Friberg, L., Norberg, G.F. and Vouk, V.B. (eds.). 2nd. Elsevier Science Publishers, Amsterdam, The Netherlands, pp. 623–637.
Bernhard, G., Geipel, G., Reich, T., Brendler, V., Amayeari, S., and Hitsche, H. (2001). Uranyl carbonate complexes formation: Validation of the Ca2UO2CO30 (aq) species. Radiochim. Acta, 89: 511–518..
Berradi, H., Betho, J.-M.., Dudoignon, N., Mazur, A., Grandcolas, L., Baudelin, C., Grison, S., Voisin, P., Gourmelon, P. and Dublineau, I. (2008). Renal anemia induced by chronic ingestion of depleted uranium in rats. Toxicol. Sci., 103(2): 397–408.
Bexfield, L.M. and Jurgens, B.C. (2014). Effects of seasonal operation on the quality of water produced by public-supply wells. Groundwater, 52(S1): 10–24.
Bhattacharyya, M.H., Larsen, R.P., Cohen, N., Ralston, L.G., Moretti, E.S., Oldham, R.D. and Ayeares, L. (1989). Gastrointestinal absorption of plutonium and uranium in fed and fasted adult baboons and mice: Application to humans. Radiat. Prot. Dosim., 26(1–4): 159–165.
Bleise, A., Danesi, P.R. and Burkart, W. (2003). Properties, use and health effects of depleted uranium (DU): A general overview. Depleted uranium. J. Environ. Radioact., 64(2–3): 93–112.
Boice, J.D., jr., Cohen, S.S., Mumma, M.T., Chadda, B. and Blot, W.J. (2008). A cohort study of uranium millers and miners of Grants, New Mexico, 1979–2005. J. Radiol. Prot., 28: 303–325.
Bosque, M.A., Domingo, J.L., Llobet, J.M. and Corbella, J. (1993). Embryotoxicity and teratogenicity of uranium in mice following subcutaneous administration of uranyl acetate. Biol. Trace Elem. Res., 36: 109–118.
Bourgeois, D., Burt-Pichat, B., LeGoff, X., Garrevoet, J., Tack, P., Falkenberg, G., Van Hoorebeke, L., Vincze, L., Denecke, M.A., Meyer, D., Vidaud, C. and Boivin, G. (2015). Micro-distribution of uranium in bone after contamination: New insight into its mechanism of accumulation into bone tissue. Anal. Bioanal. Chem., 407: 6619–6625.
Bozal, C.B., Martinez, A.B., Cabrini, R.L. and Ubios, A.M. (2005). Effect of ethane-1-hydroxy-1,1-bisphosphonate (EHBP) on endochondral ossification lesions induced by a lethal oral dose of uranyl nitrate. Arch Toxicol., 79: 475–481.
Brina, R. and Miller, A.G. (1992). Direct detection of trace levels of uranium by laser-induced kinetic phosphorimetry. Anal. Chem., 64: 1413–1418.
Briner, W. and Murray, J. (2005). Effects of short-term and long-term depleted uranium exposure on open-field behaviour and brain lipid oxidation in rats. Neurotoxicol. Teratol., 27: 135–144.
British Columbia Ministry of Environment and Climate Change Strategy (2017). Personal communication with H. Thompson.
British Columbia Ministry of Health (2015). Personal communication with D. Fishwick.
Brown, C.J., Jurgens, B.C., Katz, B.G., Landon, M.K. and and Eberts, S.M. (2007). Arsenic and uranium in four aquifer settings: Occurrence, distribution, and mechanisms for transport to supply wells. Charleston, South Carolina. 2007 NGWA Naturally Occurring Contaminants Conference: Arsenic, Radium, Radon, Uranium, March 22-23, 2007.
Brugge, D. and Buchner, V. (2011). Health effects of uranium: New research findings. Rev. Environ. Health, 26(4): 231–249.
Bussy, C., Lestaevel, P., Dhieux, B., Amourette, C., Paquet, F., Gourmelon, P. and Houpert, P. (2006). Chronic ingestion of uranyl nitrate perturbs acetylcholinesterase activity and monoamine metabolism in male rat brain. Neurotoxicology, 27(2): 245–252.
Butterworth, A. (1955). The significance and value of uranium in urine analysis. Trans. Assoc. Ind. Med. Offrs, 5(3): 36–43.
Camacho, L.M., S. Deng, and R.R. Parra. (2010) Uranium removal from groundwater by natural clinoptilolite zeolite: Effects of pH and initial feed concentration. J. Haz. Materials, 175(1–3): 393–398.
Cameco (2015). 2014 Annual compliance monitoring & operational performance report. Reporting period January 1 - December 31, 2014. Port Hope Conversion Facility. Cameco Corp., Port Hope, Ontario.
CCME (2007). Canadian soil quality guidelines for the protection of environmental and human health: Uranium. Canadian Council of Ministers of the Environment, Winnipeg, Manitoba. Available at: http://ceqg-rcqe.ccme.ca/download/en/285
CCME (2011). Scientific criteria document for the development of the Canadian water quality guidelines for the protection of aquatic life: Uranium. Canadian Council of Ministers of the Environment, PN 1451, Winnipeg, Manitoba.
CH2M Hill. (2006). Uranium and PCE treatment – Phase 1 Evaluation of treatment technologies. Technical memorandum submitted to the City of Las Cruces, New Mexico. Available at: https://donaanacounty.org/sites/default/files/pages/PhaseI-Evaluation.pdf
Chamberlain, G. (2009). India's generation of children crippled by uranium waste. In: The Guardian – International Edition, August 30th.
Chatelain, G., Bourgeois, D., Ravaux, J., Averseng, O., Vidaud, C. and Meyer, D. (2015). Incorporation of uranium into a biomimetic apatite: Physicochemical and biological aspects. J. Biol. Inorg. Chem., 20: 497–507.
Chen, J., Meyerhof, D.P. and Tracy, B.L. (2004). Model results of kidney burdens from uranium intakes. Health Phys., 86(1): 3–11.
Chen, J., Larivière, D., Timmins, R. and Verdecchia, K. (2010). Uranium GI absorption coefficient for young children. In: Proceedings of the 12th international congress of the International Radiation Protection Association (IRPA12). Buenos Aires, Argentina. International Atomic Energy Agency, Vienna, Austria.
Chen, A.S.C., Lal, V. and Wang, L. (2011a). Arsenic removal from drinking water by point of entry/point of use adsorptive media. U.S. EPA demonstration project at Oregon Institute of Technology at Klamath Falls, Oregon. Final performance evaluation report. Cincinnati, Ohio. (Report No. EPA/600/R-11/035).
Chen, J., Lariviere, D., Timmins, R. and Verdecchia, K. (2011b). Estimation of uranium GI absorption fractions for children and adults. Radiation Protection Dosimetry, 144(1–4): 379–383.
Cheng, T., Barnett, M., Roden, E. and Zhuang, J. (2004). Effects of phosphate on uranium (VI) adsorption to goethite-coated sand. Environ. Sci. Technol., 36(2): 158–165.
Clark, D.L., Neu, M.P., Runde, W., Keogh and Webster, D. (2006). Uranium and uranium compounds. In: Kirk-othmer encyclopedia of chemical technology. Uranium and uranium compounds. John Wiley & Sons, Inc.
Clifford, D.A. (1999). Ion exchange and inorganic adsorption. Chapter 9 in: Letterman, R.D.(ed). Water quality and treatment: a handbook of community water supplies, 5th edition American Water Works Association. Denver, Colorado. McGraw-Hill, New York, New York.
Clifford, D.A. (2016a). Fundamentals of radium and uranium removal from drinking water supplies. Presentation for Radionuclides Rule. Available at: www.epa.gov/dwreginfo/radionuclide-rule-presentations.
Clifford, D.A. (2016b). Personal communications. University of Houston.
Clifford, D. and Zhang, Z. (1994) Modifying ion exchange for combined radium and uranium removal, J. Am. Water Works Assoc., 86(4): 214–227.
Clifford, D.A. and Zhang, Z. (1995). Uranium and radium removal from groundwater by ion exchange resins. Chapter 1 in: Sengupta, A.K. (ed.). Ion exchange technology: advances in pollution control. Technomic Publishing Co., Lancaster, Pennsylvania.
Clifford, D., Sorg, T. and Ghurye, G. (2011). Ion exchange and adsorption of inorganic contaminants. Chapter 12 in: Edzwald, J.K. (ed.),.Water quality and treatment: a handbook of community water supplies. 6th edition. American Water Works Association,Denver, Colorado. McGraw-Hill, New York, New York.
Canadian Nuclear Safety Commission (2009). Depleted uranium: The Canadian regulator's perspective . Government of Canada. Available at: http://nuclearsafety.gc.ca/eng/resources/fact-sheets/depleted-uranium-perspective.cfm.
Canadian Nuclear Safety Commission (2012). CNSC staff report on the performance of Canadian uranium fuel cycle and processing facilities: 2011.Minister of Public Works and Government Services Canada, PWGSC Catalogue Number CC171-6/2011E; ISSN 2291-2711, Ottawa, Ontario. Available at: http://nuclearsafety.gc.ca/pubs_catalogue/uploads/CNSC-Report-Performance-Canadian-Uranium-Fuel-Cycle-Processing-Facilities-2011-eng.pdf
Canadian Nuclear Safety Commission (2014). CNSC staff report on the performance of uranium fuel cycle and processing facilities: 2012., PWGSC Catalogue Number CC171-16/2012E-PDF; ISSN 2292-6089, Ottawa, Ontario. Available at: http://www.nuclearsafety.gc.ca/pubs_catalogue/uploads/CNSC-Report-Performance-Canadian-Uranium-Fuel-Cycle-Processing-Facilities-2012-eng.pdf
Coonfare, C.T., Chen, A. and Wang. A. (2010). Arsenic and uranium removal from drinking water by adsorptive media. U.S. EPA demonstration project at Nambe Pueblo, New Mexico. Final performance evaluation report. Cincinnati, Ohio. (Report No: EPA/600/R-10/164).
Cothern, C.R. and Lappenbusch, W.L. (1983). Occurrence of uranium in drinking water in the U.S. Health Phys., 45(1): 89–99.
Dang, H.S., Pullat, V.R. and Sharma, R.C. (1995). Distribution of uranium in human organs of urban Indian population and its relationship with clearance half- lives. Health Phys., 68(3): 328–331.
Darolles, C., Broggio, D., Feugier, A., Frelon, S., Dublineau, I., De Meo, M. and Petitot, F. (2010). Different genotoxic profiles between depleted and enriched uranium. Toxicol. Lett., 192: 337–348.
De Putter, T., André, L., Bernard, A., Dupuis, C., Jedwab, J., Nicaise, D. and Perruchot, A.(2002). Trace element (Th, U, Pb, REE) behavior in a cryptokarstic halloysite and kaolinite deposit from southern Belgium: Importance of “accessory” mineral formation for radioactive pollutant trapping. Appl. Geochem., 17(10): 1313.
de Rey, B.M., Lanfranchi, H.E. and Cabrini, R.L. (1983). Percutaneous absorption of uranium compounds. Environ. Res., 30(2): 480–491.
Devarajan, P. (2010). The use of targeted biomarkers for chronic kidney disease. Adv. Chronic Kidney Dis., 17(6): 469–479.
Diamond, G.L., Morrow, P.E., Panner, B.J., Gelein, R.M. and Baggs, R.B. (1989). Reversible uranyl fluoride nephrotoxicity in the Longs Evans rat. Fundam. Appl. Toxicol., 13: 65–78.
Diaz Sylvester, P.L., Lopez, R., Ubios, A.M. and Cabrini, R.L. (2002). Exposure to subcutaneously implanted uranium dioxide impairs bone formation. Arch. Health, 57(4): 320–325.
Dinocourt, C., Legrand, M., Dublineau, I. and Lestaevel, P. (2015). The neurotoxicology of uranium. Toxicology, 337: 58–71.
Dodge, C., Francis, A., Gollow, J., Halada, G., Eng, C. and Clayton, C. (2002). Association of uranium with iron oxides typically formed on corroding steel surfaces. Environ. Sci. Technol., 36: 3504–3511.
Domingo, J.L., Llobet, J.M., Tomas, J.M. and Corbella, J. (1987). Acute toxicity of uranium in rats and mice. Bull. Environ. Contam. Toxicol., 39(1): 168–174.
Domingo, J.L., Paternain, J.L., Llobet, J.M. and Corbella, J. (1989a). The developmental toxicity of uranium in mice. Toxicology, 55(1–2): 142–152.
Domingo, J.L., Ortega, A., Paternain, J.L. and Corbella, J. (1989b). Evaluation of the perinatal and postnatal effects of uranium in mice upon oral administration. Arch. Environ. Health, 44(6): 395–398.
Dong, W. and Brook, S. (2006). Formation of aqueous Mg2UO2CO32– complex and uranium anion exchange mechanism onto an exchange resin. Environ. Sci. Technol. 42: 1979–1983.
Donnadieu-Claraz, M., Bonnehorgne, M., Dhieux, M., Maubert, C., Cheynet, M., Paquet, F. and Gourmelon, P. (2007). Chronic exposure to uranium leads to iron accumulation in rat kidney cells. Radiat. Res., 167(4): 454–464.
Drage, J. and Kennedy, G.W. (2013). Occurrence and mobilization of uranium in groundwater in Nova Scotia. In: GeoMontreal Conference Proceedings. Montreal, Quebec.
Dreesen, D.R., Williams, J.M., Marple, M.L., Gladney, E.S. and Perrin, D.R. (1982). Mobility and bioavailability of uranium mill tailings contaminants. Environ. Sci. Technol., 16(10): 702–709.
Dublineau, I., Grison, S., Baudelin, C., Dudoignon, N., Souidi, M., C., Paquet, F., Aigueperse, J. and Gourmelon, P. (2005). Absorption of uranium through the entire gastrointestinal tract of the rat. Int. J. Radiat. Biol., 81(6): 473–482.
Dublineau, I., Souidi, M., Gueguen, Y., Lestaevel, P., Bertho, J., Delissen, O., Grison, S., Paulard, A., Monin, A., Kern, Y., Rouas, C., Loyen, J., Gourmelon, P. and Aigueperse, J. (2014). Unexpected lack of deleterious effects of uranium on physiological systems following a chronic oral intake in adult rat. Biomed. Res. Int., Article ID 181989(2014. doi:10.1155/2014/181989): 24 pages.
Durbin, P.W. and Wrenn, M.E. (1976). Metabolism and effects of uranium in animals. In: Conference on occupational health experience with uranium. US Energy Research and Development Administration (ed.). NTIS, Washington, DC, ERDA-93, pp. 68–129.
EFSA (2009). Scientific opinion: Uranium in foodstuffs, in particular mineral water. Scientifi opinion of the panel on contaminants in the food chain, European Food Safety Authority. EFSA Journal, 1018: 1–59.
Eidson, A.F., Griffith, W.C.j., Hahn, F.F. and Pickrell, J.A. (1989). A model for scaling the results of U excretion rate studies in beagle dogs to man. Health Phys., 57(Sup. 1): 199–210.
Essien, I.O., Sandoval, D.N. and Kuroda, P.K. (1985). Deposition of excess amount of natural U from the atmosphere. Health Phys., 48(3): 325–331.
Favre-Reguillon, A., Lebuzit, G., Murat, D., Foos, J., Mansour, C. and Draye, M. (2008). Selective removal of dissolved uranium in drinking water by nanofiltration. Water Res., 42: 1160–1166.
Fernandez, E., Perez, R., Hernandez, A., Tejada, P., Arteta, M. and Ramos, J.T. (2011). Factors and mechanisms for pharmacokinetic differences between pediatric population and adults. Pharmaceutics, 3(1): 53–72.
Feugier, A., Frelon, S., Gourmelon, P. and Claraz, M. (2008). Alteration of mouse oocyte quality after a subchronic exposure to depleted uranium. Reprod. Toxicol., 26(3–4): 273–277.
Fisenne, I.M. and Welford, G.A. (1986). Natural U concentration in soft tissues and bone of New York city residents. Health Phys., 50(6): 739–746.
Fisenne, I.M., Perry, P.M. and Harley, N.H. (1988). Uranium in humans. Radiat. Prot. Dosim., 24(1–4): 127–131.
Focazio, M., Szabo, Z., Kraemer, T., Mullin, A., Barringer, T. and DePaul, V. (2001). Occurrence of selected radionuclides in groundwater used for drinking water in the United States: A reconnaissance survey, 1998. U.S. Department of Interior. U.S. Geological Survey. Water resources investigation report 00-4273. Available at:
https://pubs.usgs.gov/wri/wri004273/pdf/wri004273.pdf
Fox, K.R. and T.J. Sorg. (1987). Controlling arsenic, fluoride, and uranium by point-of-use treatment. J. Am. Water Works Assoc., 79(10):81–84.
Fox, P., Davis, J. and Zachara, J. (2006). The effect of calcium on aqueous uranium (VI) speciation and adsorption to ferrihydrite and quartz. Geochim. Cosmochim. Acta, 70: 1379–1387.
Frelon, S., Guipaud, O., Mounicou, S., Lobinski, R., Delissen, O., and Paquet, F. (2009). In vivo screening of proteins likely to bind uranium in exposed rat kidney. Radiochim. Acta, 97(7): 367–373.
Friedman, M.J., Hill, A.S., Reiber, S.H., Valentine, R.L., Larsen, G., Young, A., Korshin, G.V., Peng, C-Y. (2010). Assessment of inorganics accumulation in drinking water system scales and sediments. Water Research Foundation and United States Environmental Protection Agency, Denver, Colorado (Report No. 3118). Available at: www.waterrf.org/PublicReportLibrary/3118.pdf
Friedman, M., Hill, A., Booth, S., Hallett, M., McNeill, L., McLean, J., Stevens, D., Sorensen, D., Hammer, T., Kent, W., De Haan, M., MacArthur, U and Mitchell, K. (2016). Metals accumulation and release within the distribution system: Evaluation and mitigation. Water Research Foundation. Denver, Colorado (Report No:4509).
Frome, E.L., Cragle, D.L. and McLain, R.W. (1990). Poisson regression analysis of the mortality among a cohort of World War II nuclear industry workers. Radiat. Res., 123(2): 138–152.
Fukuda, S., Ikeda, M., Chiba, M. and Kaneko, K. (2006). Clinical diagnostic indicators of renal and bone damage in rats intramuscularly injected with depleted uranium. Radiat. Prot. Dosimetry, 118(3): 307–314.
Fuller, C.C., Barger, J.R., Davis, J.A. and Piana, M.J. (2002). Mechanisms of uranium interactions with hydroxyapatite: implications for groundwater remediation. Environ. Sci. Technol. 36: 158–165.
Garbarino, J.R. and Struzeski, T.M. (1998). Methods of analysis by the U.S. Geological Survey National Water Quality Laboratory -Determination of elements in whole-water digest using inductively coupled plasma-optical emission spectrometry and inductively coupled plasma-mass spectrometry. United States Geological Survey, Denver, Colorado (Report No. 98-165). Available at: http://nwql.usgs.gov/pubs/OFR/OFR-98-165.pdf
Gilkeson, R.H., Cartwright, K. Cowart, J.B., and Holtzman, R.B. (1983). Hydrogeologic and geochemical studies of selected natural radioisotopes and barium in groundwater in Illinois. Illinois Department of Energy and Natural Resources. Champaign, Illinois.Available at: https://ia802700.us.archive.org/8/items/hydrogeologicgeo19836gilk/hydrogeologicgeo19836gilk.pdf
Gilman, A.P., Villeuve, D.C., Secours, V.E., Yagminas, A.P., Tracy, B.L., Quinn, J.M., Valli, V.E., Willes, R.J. and Moss, M.A. (1998a). Uranyl nitrate: 28-day and 91-day toxicity studies in the Sprague-Dawley rat. Toxicol. Sci., 41(1): 117–128.
Gilman, A.P., Villenuve, D.C., Secours, V.E., Yagminas, A.P., Tracy, B.L., Quinn, J.M., Valli, V.E. and Moss, M.A. (1998b). Uranyl nitrate: 91-day toxicity studies in the New Zealand white rabbit. Toxicol. Sci., 41(1): 129–137.
Gilman, A.P., Moss, M.A., Villenuve, D.C., Secours, V.E., Yagminas, A.P., Tracy, B.L., Quinn, J.M., Long, G. and Valli, V.E. (1998c). Uranyl nitrate: 91-day exposure and recovery studies in the male New Zealand white rabbit. Toxicol. Sci., 41(1): 138–151.
Gilson, E.R., Huang, S., Koster van Groos, P.G., Schechel, K.G., Qafoku, O., Peacock, A.D., Kaplan, D.I. and Jaffé, P.R. (2015). Uranium redistribution due to water table fluctuations in sandy wetland mesocosms. Environ. Sci. Technol., 49(20): 12214–12222.
Ginder -Vogel, M., Criddle, C. and Fendorf, S. (2006). Thermodynamic constraints on the oxidation of biogenic UO2 by Fe(III) (hydr)oxides. Environ. Sci. Technol. 40: 3544–3550.
Gizyn, W. (1994). Windsor air quality study: soil and garden produce survey results. Phytotoxicology Section, Standards Development Branch, Ontario Ministry of Environment and Energy, Windsor, ON.
Goel, K.A., Garg, V.K. and Garg, W. (1979). Histopathological alterations induced by uranyl nitrate in the liver of albino rat. Bull. Environ. Contam. Toxicol., 22(1): 785–790.
Gordon, S. (1992). Link between ore bodies and biosphere concentrations of uranium. Atomic Energy Control Board of Canada, ACEB Project No. 5.140.1, Ottawa, Canada.
Grafvert, T., C. Ellmark, and E. Holm. (2002). Removal of radionuclides at a waterworks. J. Environ. Radioact., 63: 105–115.
Grison, S., Favé, G., Maillot, M., Manens, L., Delissen, O., Blancherdon, E., Banzet, N., Bott, R. and Dublineau, I. (2013). Metabolomics identifies a biological response to chronic low-dose natural uranium contamination in urine samples. Metabolomics, 9: 1168–1180.
Gueguen, Y., Rouas, C., Monin, A., Manens, L., Stefani, J., Delissen, O., Grison,, S. and Dublineau, I. (2014). Molecular, cellular, and tissue impact of depleted uranium on xenobiotic-metabolizing enzymes. Arch. Toxicol., 88: 227–239.
Guglielmotti, M.B., Ubios, A.M., de Rey, B.M. and Cabrini, R.L. (1984). Effects of acute intoxication with uranyl nitrate on bone formation. Experientia, 40: 474–476.
Guglielmotti, M.B., Ubios, A.M. and Cabrini, R.L. (1985). Alveolar wound healing alteration under uranyl nitrate intoxication. J. Oral Path., 14: 565–572.
Guseva Canu, I., Jacob, S., Cardis, E., Wild, P., Caër, S., Auriol, B., Garsi, J.P., Tirmarche, M. and Laurier, D. (2011). Uranium carcinogenicity in humans might depend on the physical and chemical nature of uranium and its isotopic composition: Results from pilot epidemiological study of French nuclear workers. Cancer Cause Control, 22: 1563–1573.
Gustafsson, J., Dässman, E., Bäckström, M. (2009). Towards a consistent geochemical model for prediction of uranium (VI) removal from groundwater by ferrihydrite. Appl. Geochem., 24: 454–462.
Hamid, W.J. (2012). Uranyl nitrate induce histological changes in the kidney. Int. J. Eng. Technol,, 12(4): 9–13.
Hanson, S.K., Wilson, D.B.and Gunaji, N.,N.. (1987). Removal of uranium from drinking water by ion exchange and chemical clarification. EPA/600/S2-87/076. Cincinnati, Ohio.
Hao, Y., Li, R., Leng, Y., Ren, J., Liu, J., Ai, G., Xu, H., Su, Y. and Cheng, T. (2009). A study assessing the genotoxicity in rats after chronic oral exposure to a low dose of depleted uranium. J. Radiat. Res., 50: 521–528.
Hao, Y., Ren, J., Liu, J., Yang, Z., Liu, C., Li, R. and Su, Y. (2013a). Immunological changes of chronic oral exposure to depleted uranium in mice. Toxicology, 309(0): 81–90.
Hao, Y., Ren, J., Liu, J., Yang, Z., Liu, C., Li, R. and Su, Y. (2013b). Immunological changes of chronic oral exposure to depleted uranium in rats. Health Phys., 105(3): 3–10.
Harduin, J.C., Royer, P. and Piechowski, J. (1994). Uptake and urinary excretion of uranium after oral administration in man. Radiat. Prot. Dosim., 53(1–4): 245–248.
Harrison, J.D. and Stather, J.W. (1981). The gastrointestinal absorption of proactinium, uranium and neptunium in the hamster. Radiat. Res., 88: 47–55.
Haynes, W.M. (2014). Handbook of chemistry and physics. Chapter 4 – The elements. pp.4-39–4-40. 95th. CRC Press, Boca Raton, Florida .
Health Canada (1994). Canadian Environmental Protection Act. Human health risk assessment for priority substances. Minister of Supply and Services Canada, Ottawa, Ontario.
Health Canada (1998 ). Assessment of the effect on kidney function of long-term ingestion of uranium in drinking water by the Kitigan Zibi Community. Report to Medical Services Branch on a Study Conducted by the Radiation Protection Bureau, Health Protection Branch.
Health Canada (2009). Guidelines for Canadian drinking water quality: guideline technical document — radiological parameters. Radiation Protection Bureau, Healthy Environments and Consumer Safety Branch, Health Canada, Ottawa, Ontario. Available at: http://healthycanadians.gc.ca/publications/healthy-living-vie-saine/water-radiological-radiologique-eau/index-eng.php
Health Canada (2010). Report on human biomonitoring of environmental chemicals in Canada: results of the Canadian health measures survey cycle 1 (2007 – 2009). Ottawa, Ontario. Available at: www.hc-sc.gc.ca/ewh-semt/pubs/contaminants/chms-ecms/index-eng.php
Health Canada (2011). Canadian total diet study (2001 – 2007), dietary intakes of contaminants & other chemicals for different age-sex groups of Canadians. Bureau of Chemical Safety, Health Products and Foods Branch, Ottawa, Ontario. Available at: www.hc-sc.gc.ca/fn-an/surveill/total-diet/intake-apport/index-eng.php.
Health Canada (2014). Canadian guideline for the management of naturally occurring radioactive materials (NORM). Prepared by the Canadian working group of the Federal Provincial Territorial Radiation Protection Committee. Ottawa, Ontario. Available at: www.hc-sc.gc.ca/ewh-semt/alt_formats/pdf/pubs/contaminants/norm-mrn/norm-mrn-eng.pdf
Health Canada (2013). Second report on human biomonitoring of environmental chemicals in Canada: Results of the Canadian Health Measures Survey cycle 2 (2009 – 2011). Available from: www.hc-sc.gc.ca/ewh-semt/pubs/contaminants/chms-ecms-cycle2/index-eng.php
Health Canada (2015a). Total diet study. 1993 - 2012. Personal communication with Dabeka, R.
Health Canada (2015b). Personal communication with B. Jessiman, Healthy Environments and Consumer Safety Branch.
Health Canada (2015c). Third report on human biomonitoring of environmental chemicals in Canada: Results of the Canadian health measures survey cycle 3 (2012 – 2013). Available at: www.hc-sc.gc.ca/ewh-semt/pubs/contaminants/chms-ecms-cycle3/index-eng.php
Health Canada (2015d). Personal communication with A.M. Tugulea, Environmental and Radiation Health Sciences Directorate.
Hedge, A.M., Kar, A. and Shetty, Y.R. (2016). Urine analysis of children residing near uranium mines. Int. J. Community Med. Public Health, 3(5): 1112–1114.
Hess, C.T., Michel, J., Horton, T.R., Prichard, H.M. and Coniglio, W.A. (1985). The occurrence of radioactivity in public water supplies in the United States. Health Physics, 48(5): 553–586.
Hoet, P., Deumer, G., Bernard, A., Lison, D. and Haufroid, V. (2015). Urinary trace element concentrations in environmental settings: is there a value for systematic creatinine adjustment or do we introduce a bias? J Expo Sci Environ Epidemiol., April 1: 1–7.
Höllriegl, V., Arogunjo, A.M., Giussani, A., Michalke, B. and Oeh, U. (2011). Daily urinary excretion of uranium in members of the public of Southwest Nigeria. Sci. Total Environ., 412–413: 344–350.
Houpert, P., Lestaevel, P., Bussy, C., Paquet, F., and Gourmelon, P. (2005). Enriched but not depleted uranium affects central nervous system in long-term exposed rat. Neurotoxicology, 26, 1015–1020.
Huikuri, P., Salonen, L.and Raff, O.(1998). Removal of natural radionuclides from drinking water by point of entry reverse osmosis. Desalination, 119(1–3): 235–239.
Hursh, J.B., Neuman, W.R., Toribara, T., Wilson, H. and Waterhouse, C. (1969). Oral ingestion of uranium by man. Health Phys., 17: 619–621.
Huxstep, M.R. and Sorg, T.J. (1988). Removal of inorganic contaminants by reverse osmosis pilot plants. Cincinnati, Ohio. (Report No. EPA/600/S2-87/109).
IARC (1999). IARC monographs on the evaluation of carcinogenic risks to humans: Surgical implants and other foreign bodies. Volume 74. International Agency for Research on Cancer IARC Working Group on the Evaluation of Carcinogenic Risks to Humans (ed.). IARC Press, Lyons, France. 427 pp.
ICRP (1995). Uranium. Internal Commission on Radiological Protection. Ann. ICRP, 25(1): 57–74. Doi: 10.1016/0146-6453(95)90059-4.
ICRP (2012). Compendium of dose coefficients based on ICRP publication 60. Internal Commission on Radiological Protection ICRP publication 119. Ann. ICRP, 41(Suppl.): 1–130.
Israelsson, A., and Pettersson, H. (2014). Measurements of 234U and 238U in hair, urine, and drinking water among drilled bedrock well water users for the evaluation of hair as a biomonitor of uranium intake. Health Phys., 107(2): 143–149.
Jacques, D., Mallants, D., Simunek, J. and Th. van Genuchten, M. (2008). Modelling the fate of uranium from inorganic phosphorus fertilizer applications in agriculture. In: Loads and fate of fertilizer-derived uranium. De Kok, L.J. and Schnug, E. (eds.). Backhuys Publishers, Leiden, Netherlands. pp. 57–64.
Jelinek, R.T. and Correll, R.J. (1987). Operation of small scale uranium removal systems radionuclides. In: Proceedings of American Water Works Association seminar on radionuclides in drinking water. American Water Works Association Annual Conference, Kansas City, Missouri. American Water Works Association, Denver, Colorado.
Jelinek, R.T. and Sorg, T.G. (1988). Operating a small full-scale ion exchange system for uranium removal. J. Am. Water Works Assoc., 80(7): 79.
Jiang, G., Tidwell, K., McLaughlin, B.A., Cai, J., Gupta, R., Milatovic, D., Nass, R. and Aschner, M. (2007). Neurotoxic potential of depleted uranium - effects in primary cortical neuron cultures and in Caenorhabditis elegans. Toxicol. Sci., 99(2): 553–565.
Joksić, A.S., and Katz, S.A. (2014). Efficacy of hair analysis for monitoring exposure to uranium: A mini-review. J Environ Sci Health A Tox. Hazard. Subst. Environ. Eng., 49(13): 1578–1587.
Jurgens, B., Fram, M., Belitz, B., Burow,K., and Landon, M. (2010). Effects of groundwater development on uranium: Central Valley, California, USA. Ground Water, 48(6): 913–928.
Kalmykov, S. and Choppin, G. (2000). Mixed Ca2+/UO22+/Co32– complex formation at different ionic strength. Radiochim. Acta., 88: 603–606.
Kar, A., Shetty, Y.R. and Hedge, A.M. (2016). Enamel defects seen among children residing near uranium mines. A case control study. Iosr-Jdms, 15(1): 137–142.
Karpas, Z., Lorber, A., Elish, E., Kol, R., Roiz, Y., Marko, R., Katorza, E., Halicz, L., Riondato, J., Vanhaecke, F. and Moens, L. (1998). Uptake of ingested uranium after low "acute intake". Health Phys., 74(3): 337–345.
Karpas, Z., PazTal, O., Lorber, A., Salonen, L., Komulainen, H., Auvinen, A., Saha, H. and Kurttio, P. (2005). Urine, hair, and nails as indicators for ingestion of uranium in drinking water. Health Phys., 88(3): 229–242.
Kathren, R.L. and Burklin, R.K. (2008). Acute chemical toxicity of uranium. Health Phys., 94(2): 170–179.
Katsoyiannis, I.A. (2007) Carbonate effects and pH-dependence of uranium sorption onto bacteriogenic iron oxides: Kinetic and Equilibrium Studies. J. Haz. Materials., 39: 31–37.
Keith, L.S., Faroon, O.M. and Fowler, B.A. (2007). Chapter 45 - Uranium. In: Handbook on the toxicology of metals (third edition). Nordberg, G.F., Fowler, B.A., Nordberg, M. and Friberg, L.T. (eds.). 3rd. Academic Press, Burlington, pp. 881–903.
Kelada, I., Osman, A., Fouad, G. and Radi, S. (2008). Experimental simulation for accidental exposure to uranium pollutants in drinking water. A histological study on the possible impact to the cerebral cortex of adult albino rats. Bull. Alex. Fac. Med., 44(1): 253–260.
Konietzka, R. (2015). Gastrointestinal absorption of uranium compounds – A review. Regul. Toxicol. Pharm., 71(1): 125–133.
Krishnan, K. and Carrier, R. (2008). Approaches for evaluating the relevance of multiroute exposures in establishing guideline values for drinking water contaminants. J. Environ. Sci. Health C, 26(3): 300–316.
Krishnan, K. and Carrier, R. (2013). The use of exposure source allocation factor in the risk assessment of drinking-water contaminants. J. Toxicol. Environ. Health B, 16(1): 39–51.
Kundt, M.S., Martinez-Taibo, C., Muhlmann, M.C. and Furnari, J.C. (2009). Uranium in drinking water: Effects on mouse oocyte quality. Health Phys., 96(5): 568–574.
Kurttio, P., Auvinen, A., Salonen, L., Saha, H., Pekkanen, J., Mäkeläinen, I., Väisänen, S.B., Penttilä, I.M. and Komulainen, H. (2002). Renal effects of uranium in drinking water. Environ. Health Perspect., 110(4): 337–342.
Kurttio, P., Komulainen, H., Leino, A., Salonen, L., Auvinen, A. and Saha, H. (2005). Bone as a possible target of chemical toxicity of natural uranium in drinking water. Environ. Health Perspect., 113(1): 68–72.
Kurttio, P., Harmoinen, A., Saha, H., Salonen, L., Karpas, Z., Komulainen, H. and Auvinen, A. (2006a). Kidney toxicity of ingested uranium from drinking water. Am J Kidney Dis., 47(6): 972–982.
Kurttio, P., Salonen, L., Ilus, T., Pekkanen, J., Pukkala, E. and Auvinen, A. (2006b). Well water radioactivity and risk of cancers of the urinary organs. Environ. Res., 102(3): 333–338.
La Touche, Y.D., Willis, D.L. and Dawydiak, O.I. (1987). Absorption and biokinetics of U in rats following an oral administration of uranyl nitrate solution. Health Phys., 53(2): 147–162.
Lack, J.G., Chaudhuri, S.K., Chakraborty, R., Achenbach, L.A. and Coates, J.D. (2002). Anaerobic biooxidation of fe(II) by dechlorosoma suillum. Microb. Ecol., 43(4): 424-431.
Landa, E.R. and Councell, T.B. (1992). Leaching of uranium from glass and ceramic foodware and decorative items. Health Phys., 63(3): 343–348.
Lane, R., Thompson, P., Ilin, M., Phaneuf, M., Burtt, J. and Reinhardt, P. (2011). Use of weight of evidence approach to determine the likelihood of adverse effects on human health from the presence of uranium facilities in Port Hope, Ontario. J. Environ. Prot., 2: 1149–1161.
Langmuir, D. (1978). Uranium solution –mineral equilibrium at low temperatures with applications to sedimentary ore deposits. Geochim. Cosmochim. Acta, 42: 547–569.
Langmuir, D. (1997). Actinides and their daughter and fission products. Chapter 13 in: Langmuir, D. (ed.). Aqueous environmental geochemistry. Prentice-Hall Inc., Upper Saddle River, N.J. ISBN 0023674121.
Larivière, D., Packer, A.P., Marro, L., Li, C., Chen, J. and Cornett, R.J. (2007). Age dependence of natural uranium and thorium concentrations in bone. Health Phys., 92(2): 119–126.
Larivière, D., Tolmachev, S.Y., Kochermin, V. and Johnson, S. (2013). Uranium bone content as an indicator of chronic environmental exposure from drinking water. J. Environ. Radioact., 121: 98–103.
Larsen, R.P., Bhattacharyva, M.H., Oldham, R.D., Moretti, E.S. and Cohen, N. (1984). Gastrointestinal absorption and retention of plutonium and uranium in the baboon. In: Environmental research division annual report July 1982 - 1983. ANL-83-100-Pt2. Argonne National Laboratory, Argonne, Illinois, pp. 51–60.
Lassovszky, P. and Hathaway, S.W. (1983). Treatment technologies to remove radionuclides from drinking water. Pre-conference reportfor national workshop on radioactivity in drinking water. U.S. EPA, Washington, DC. Lee, M. (2009). Basic skills in interpreting laboratory data. Lee, M. (ed.). 4th. American Society of Health-System Pharmacists, Bethesda, Maryland.
Lee, S.Y. and Bondietti, E. (1983). Removing uranium from drinking water by metal hydroxides and anion exchange resin. J. Am. Water Works Assoc., 75(10): 536.
Lee, S., Hall, S. and Bondietti, E. (1982). Methods of removing uranium from drinking water: 2. Present municipal water treatment and potential removal methods. Washington, DC. (Report No: EPA 570/9-82/003).
Leggett, R.W. (1989). The behavior and chemical toxicity of U in the kidney: A reassessment. Health Phys., 57(3): 365–383.
Leggett, R.W. and Harrison, J.D. (1995). Fractional absorption of ingested uranium in humans. Health Phys., 68(4): 484–498.
Lestaevel, P., Bussy, C., Paquet, F., Dhieux, B., Clarençon, D., Houpert, P. and Gourmelon, P. (2005). Changes in sleep–wake cycle after chronic exposure to uranium in rats. Neurotoxicol. Teratol., 27(6): 835–840.
Lewis, G.M., A.S.C. Chen, A.S.C. and Li. Wang, L.. (2007). Arsenic removal from drinking water by Point of Use Reverse Osmosis (POU RO). U.S. EPA demonstration project at Sunset Ranch development in Homedale, ID. Final performance evaluation report. Cincinnati, Ohio. (Report No: EPA 600/R-07/082).
Limson Zamora, M., Tracy, B.L., Zielinski, J.M., Meyerhof, D.P. and Moss, M.A. (1998). Chronic ingestion of uranium in drinking water: A study of kidney bioeffects in humans. Toxicol. Sci., 43(1): 68–77.
Limson Zamora, M., Tracy, B., Zielinski, J.M., Meyerhof, D.P., Moodie, G.B. and Falcomer, R. (2002a). Uranium gastrointestinal absorption in humans: A chronic exposure study. Health Canada, Radiation Protection Bureau, Ottawa, Canada. Available at: www.irpa.net/irpa10/cdrom/00783.pdf.
Limson Zamora, M., Zielinski, J.M., Meyerhof, D.P. and Tracy, B.L. (2002b). Gastrointestinal absorption of uranium in humans. Health Phys., 83(1): 35–45.
Limson Zamora, M., M. Zielinski, J., Meyerhof, D., Moodie, G., Falcomer, R. and Tracy, B. (2003). Uranium gastrointestinal absorption: The F1 factor in humans. Radiat. Prot. Dosimetry, 105(1–4): 55–60.
Limson Zamora, M., Zielinski, J.M., Moodie, G.B., Falcomer, R.A., Hunt, W.C. and Capello, K. (2009). Uranium in drinking water: Renal effects of long-term ingestion by an aboriginal community. Arch. Environ. Occup. Health, 64(4): 228–241.
Lin, R.H., Lih, J.W., Lee, C.H. and Lin-Shiau, S.Y. (1993). Cytogenetic toxicity of uranyl nitrate in Chinese hamster ovary cells. Mutat. Res., 319: 197–203.
Lin, Z.Q., Hendershot, W.H., Kennedy, G.G., Dutilleul, P. and Schuepp, P.H. (1996). Total elements in forest soils affected by acid deposition in southern Quebec. Can. J. Soil. Sci., 76(2): 165–171.
Linares, V., Albina, M.L., Bellés, M., Mayayo, E., Sánchez, D.J. and Domingo, J.L. (2005). Combined action of uranium and stress in the rat: II. Effects on male reproduction. Toxicol. Lett., 158(3): 186–195.
Linares, V., Bellés, M., Albina, M.L., Sirvent, J.J., Sánchez, D.J. and Domingo, J.L. (2006). Assessment of the pro-oxidant activity of uranium in kidney and testis of rats. Toxicol. Lett., 167(2): 152–161.
Linares, V., Sánchez, D.J., Bellés, M., Albina, L., Gómez, M. and Domingo, J.L. (2007). Pro-oxidant effects in the brain of rats concurrently exposed to uranium and stress. Toxicology, 236(1–2): 82–91.
Ljung Bjorklund, K., Vahter, M., Palm, M., Lignell, S. and Berglund, M. (2012). Metals and trace element concentrations in breast milk of first time healthy mothers: A biological monitoring study. Environ. Health, 11: 92.
Llobet, J.M., Sirvent, J.J., Ortega, A. and Domingo, J.L. (1991). Influence of chronic exposure to uranium on male reproduction in mice. Fundam. Appl.Toxicol., 16(4): 821–829.
Loomis, D.P. and Wolf, S.H. (1996). Mortality of workers at a nuclear materials production plant at Oak Ridge, Tennessee, 1947?1990. Am. J. Ind. Med., 29(2): 131–141.
Lowry, J., (2009). Lakhurst Acres, ME: Compliance issues engineering problems and solutions. U.S. EPA Sixth annual drinking water workshop,. Cincinatti, Ohio.
Lowry, J., (2010). Corrosion control with air stripping. American Water Work Association Inorganic contaminants workshop. American Water Works Association, Denver, Colorado.
Lytle, D., Sorg, T., Wang, L., and Chen, C. (2014). The accumulation of radioactive contaminants in drinking water distribution systems. Water Res., 50: 396–407
Magdo, H.S., Forman, J., Graber, N., Newman, B., Klein, K., Satlin, L., Amler, R.W., Winston, J.A. and Landrigan, P.J. (2007). Grand Rounds: Nephrotoxicity in a young child exposed to uranium from contaminated well water. Environ. Health Perspect., 115(8): 1237–1241.
Malcom Pirnie Inc. (2009). Colorado radionuclide abatement and disposal strategy (CO-RADS): Bench and pilot testing report. Report submitted to Colorado Department of Health and Environment, Denver, Colorado.
Mao, Y., Desmeules, M., Schaubel, D., Berube, D., Dyck, R., Brule, D. and Thomas, B. (1995). Inorganic components of drinking water and microalbuminuria. Environ. Res., 71(2): 135–140.
Manitoba Sustainable Development (2018). Personal communication with K. Philip, Office of Drinking Water.
Maynard, E.A. and Hodge, H.C. (1949). Studies of toxicity of various uranium compounds when fed to experimental animals. In: Pharmacology and toxicology of uranium compounds: With a section on the pharmacology and toxicology of fluorine and hydrogen fluoride. Voegtlin, C. and Hodge, H.C. (eds.). Division VI- Volume 1. McGraw-Hill Book Company, Inc., New York, pp. 309–376.
Maynard, E.A., Downs, W.L. and Hodge, H.C. (1953). Oral toxicity of uranium compounds. In: Pharmacology and toxicology of uranium compounds. Voegtlin, C. and Hodge, H.C. (eds.). McGraw-Hill, New York, NY, pp. 1121–1369.
McDermott, R., Johnson, H., Staines, A., Boran, G., Sayers, G., Keogh, J. and O'Herlihy (2005). Uranium in drinking water: The Baltinglass study. Epidemiology, 16(5): S68–S69.
McDiarmid, M.A., Engelhardt, S.M., Oliver, M., Gucer, P., Wilson, P.D., Kane, R., Cernich, A., Kaup, B., Anderson, L., Hoover, D., Brown, L., Albertini, R., Gudi, R., JacobsonKram, D. and Squibb, K.S. (2007). Health surveillance of Gulf War I veterans exposed to depleted uranium: updating the cohort. Health Phys., 93(1): 60–73.
McDiarmid, M.A., Engelhardt, S., Oliver, M., Gucer, P., Wilson, P.D., Kane, R., Kabat, M., Kaup, B., Anderson, L., Hoover, D., Brown, L., Handwerger, B., Albertini, R.J., Jacobson-Kram, D., Thorne, C.D. and Squibb, K.S. (2004). Health effects of depleted uranium on exposed Gulf War veterans: A 10-year follow-up. J. Toxicol. Environ. Health A, 67(4): 277–296.
McDiarmid, M.A., Engelhardt, S.M., Dorsey, C.D., Oliver, M., Gucer, P., Wilson, P.D., Kane, R., Cernich, A., Kaup, B., Anderson, L., Hoover, D., Brown, L., Albertini, R., Gudi, R. and Squibb, K.S. (2009). Surveillance results of depleted uranium–exposed Gulf War I veterans: Sixteen years of follow-up. J. Toxicol. Environ. Health A, 72(1): 14–29.
McDiarmid, M.A., Gaitens, J.M. and Squibb, K.S. (2012). Chapter 20: Uranium and thorium. In: Patty's toxicology. Bingham, E. and Cohrssen, B. (eds.). 6th, Volume 1. John Wiley & Sons, Inc., pp. 769–816.
McDonald-Taylor, C.K., Bhatnagar, M.K. and Gilman, A. (1992). Uranyl nitrate-induced glomerular basement membrane alterations in rabbits: A quantitative analysis. Bull. Environ. Contam. Toxicol., 48: 367–373.
McDonald-Taylor, C.K., Singh, A. and Gilman, A. (1997). Uranyl nitrate-induced proximal tubule alterations in rabbits: A quantitative analysis. J. Toxicol. Pathol., 25(4): 381–389.
McGeoghegan, D. and Binks, K. (2000). The mortality and cancer morbidity experience of workers at the Capenhurst uranium enrichment facility 1946-95. J. Radiol. Prot., 20: 381–401.
Milgram, S., Carrière, M., Thiebault, C., Malaval, L. and Gouget, B. (2008). Cytotoxic and phenotypic effects of uranium and lead on osteoblastic cells are highly dependent on metal speciation. Toxicology, 250(1): 62–69.
Miller, A.C. (2009). Depleted uranium: Toxicology and health consequences. In: General, applied and systems toxicology. John Wiley & Sons, Ltd. DOI: 10.1002/9780470744307.gat136.
Miller, A.C., Stewart, M. and Rivas, R. (2010). Preconceptional paternal exposure to depleted uranium: transmission of genetic damage to offspring. Health Phys., 99(3): 371–379.
Ministère du Développement durable, de l'Environnement et de la Lutte contre les changements climatiques du Québec, Direction des eaux municipales (2015). Personal communication with C. Robert.
Morrow, P., Gelein, R., Beiter, H., Scott, J., Picano, J. and Yuile, C. (1982). Inhalation and intravenous studies of UF6/UO2F2 in dogs. Health Phys., 43(6): 859–873.
Moss, M.A. (1985). Chronic low level uranium exposure via drinking water- clinical investigations in Nova Scotia. M.Sc. thesis. Dalhousie University, Halifax, NS.
Moss, M.A., McCurdy, R.F., Dooley, K.C., Givner, M.L., Dymond, L.C., Slayter, J.M. and Courneya, M.M. (1983). Uranium in drinking water - Report on clinical studies in Nova Scotia. In: Chemical toxicology and clinical chemistry of metals. Brown, S.S. and Savory, J. (eds.). Academic Press, London, pp. 149–152.
Muikku, M., Puhakainen, M., Heikkinen, T. and Ilus, T. (2009). The mean concentration of uranium in drinking water, urine, and hair of the occupationally unexposed Finnish working population. Health Phys., 96(6): 646–654.
Müller, C. and Ruzicka-Jaroslav Bakstein, L. (1967). The sex ratio in the offspring of uranium. Acta Univ.Carol. Med., 13: 599–603.
Murray, K.K., Boyd, R.K., Eberlin, M.N., Langley, G.J., Li, L. and Naito, Y. (2013). Definitions of terms relating to mass spectrometry (IUPAC recommendations 2013). Pure and Appl. Chem., 85(7): 1515–1609.
National Research Council (1988). Health risks of radon and other internally deposited alpha-emitters: BEIR IV. Health risks of radon and other internally deposited alpha-emitters: BEIR IV. The National Academies Press, Washington, DC.
National Research Council (1999). Health effects of exposure to radon: BEIR VI. Health effects of exposure to radon: BEIR VI. The National Academies Press, Washington, DC.
Natural Resources Canada (2014). About uranium. Government of Canada, Available at: www.nrcan.gc.ca/node/7695.
NCRPM (1984). Exposure from uranium series with emphasis on radon and its daughters. National Council on Radiation Protection and Measurements, NCRP Report No. 77, Bethesda, Maryland.
Nevin, K.P. and Lovley, D.R. (2000). Potential for nonenzymatic reduction of fe(III) via electron shuttling in subsurface sediments. Environ. Sci. Technol., 34(12): 2472–2478.
New Brunswick Department of Health (2015). Personal communication with K. Gould.
Newfoundland and Labrador Department of Environment and Conservation - Water Resources Management Division (2015). Personal communication with Ferris, S.
Nolan, J. and Webber, K.A. (2015). Natural uranium contamination in major U.S. aquifers linked to nitrate. Environ. Sci. Technol. Lett., 2: 215–220.
Norrstrom, A. and Lov, A. (2014). Uranium theoretical speciation for drinking water from private drilled wells in Sweden – Implications for choice of removal method. Appl. Geochem., 51: 148–154.
Nova Scotia Environment - Drinking Water Science Division (2015). Personal communication with C. Mosher.
NSF/ANSI (2016). Drinking water system components—lead content. NSF International/American National Standards Institute, Ann Arbor, Michigan.
NSF/ANSI (2017a). Standard 60 – Drinking water treatment chemicals-health effects. NSF International/American National Standards Institute, Ann Arbor, Michigan.
NSF/ANSI (2017b). Standard 61: Drinking water system components—health effects. NSF International/American National Standards Institute, Ann Arbor, Michigan.
NSF/ANSI (2017c). In: NSF/ANSI Standard 58: Reverse osmosis drinking water treatment systems Section 7.. NSF International, Ann Arbor, Michigan.
Nunavut Department of Health (2015). Personal communication with M. LeBlanc-Havard.
OEHHA (2001). Public health goal for uranium in drinking water. Office of Environmental Health Hazard Assessment, California Environmental Protection Agency.
Ontario Ministry of the Environment and Climate Change (2015). Personal communication with S. Deshpande.
Orcutt, J.A. (1949). The toxicology of compounds of uranium following applications to the skin. In: Pharmacology and toxicology of uranium compounds: With a section on the pharmacology and toxicology of fluorine and hydrogen fluoride. Voegtlin, C. and Hodge, H.C. (eds.). Division VI - Volume 1. McGraw-Hill Book Company, Inc., New York, pp. 377–414.
Orloff, K.G., Mistry, K., Charp, P., Metcalf, S., Marino, R., Shelly, T., Melaro, E., Donohoe, A.M. and Jones, R.L. (2004). Human exposure to uranium in groundwater. Environ. Res., 94(3): 319–326.
Ortega, A., Domingo, J.L., Llobet, J.M., Tomas, J.M. and Paternain, J.L. (1989). Evaluation of the oral toxicity of uranium in a 4-week drinking water study in rats. Bull. Environ. Contam. Toxicol., 42: 935–941.
Osman, A., Geipel, G., Barkleit, A. and Bernhard, G. (2015). Uranium(VI) binding forms in selected human body fluids: Thermodynamic calculations versus spectroscopic measurements. Chem. Res. Toxicol., 28: 238–247.
Painter, S., Cvetkovic V., Pickett D. and Turner D.R. (2002). Significance of kinetics for sorption on inorganic colloids: Modeling and experiment interpretation issues. Environ. Sci. Technol., 36(24): 5369.
Pannu, T.S., Sukhmani and Karamjit, S.G. (2015). Uranium-toxicty and uranium-induced osteoscarcoma using a new regimen and surgery: A fist-time experience. J. Clin. Diagn. Res., 9(6): RD01–RD03.
Paquet, F., Houpert, P., Blancherdon, E., Delissen, O., Maubert, C., Dhieux, B., Moreels, A.M., Frelon, S., Voisin, P. and Gourmelon, P. (2006). Accumulation and distribution of uranium in rats after chronic exposure by ingestion. Health Phys., 90(2): 139–147.
Paternain, J.L., Domingo, J.L., Ortega, A. and Llobet, J.M. (1989). The effects of uranium on reproduction, gestation, and postnatal survival in mice. Ecotoxicol. Environ. Safety, 17(3): 291–296.
Patocka, J. (2014). Human health and environmental uranium. Mil. Med. Sci. Lett. (Voj. Zdrav. Listy), 83(3): 120–131.
Pawlak, Z. and Rabiega, G. (2002). Comparison of inductively coupled plasma-mass spectrometry and radiochemical techniques for total uranium in environmental water samples. Environ. Sci. Technol., 36: 5395–5398.
Pelayo, J.C., Andrews, P.M., Coffey, A.K., Calcogno, P.L., Eisner, G.M. and Jose, P.A. (1983). The influence of age on acute renal toxicity of uranyl nitrate in dogs. Pediatr. Res., 17(12): 985–992.
Pellmar, T., Fuciarelli, A.F., Ejnik, E.J., Hamilton, M., Hogan, J., Strocko, S., Emond, C., Mottaz, H.M. and Landauer, M.R. (1999). Distribution of uranium in rats implanted with depleted uranium pellets. Toxicol. Sci., 49: 29–39.
Peng, C-Y., Korshin, G.V. (2011). Speciation of trace inorganic contaminants in corrosion scales and deposits formed in drinking water distribution systems. Water Res. 45: 5553–5563.
Persello, J. (2000). Surface and interface structure of silicas. Chapter 10 in: Papirer, E. (ed.). Adsorption on Silica Surfaces. Marcel Dekker, Inc., New York, New York.
Phillips, S., Burow, K., Rewis, D. Shelton, J. and Jurgens, B. (2007). Hydrogeologic setting and groundwater flow simulations of the San Joaquin Valley regional study area, California. Section 4 in: Paschke, S. (ed.). Hydrogeologic settings and ground water flow simulations for regional studies of the transport of anthropogenic and natural contaminants to public-supply wells–studies begun in 2001. USGS Professional Paper 1737-A. Reston, Virginia. Available at: http://pubs.usgs.gov/pp/2007/1737a/.
Pilgrim, W. and Schroeder, B. (1997). Multi-media concentrations of heavy metals and major ions from urban and rural sites in new brunswick, canada. Environ. Monit. Assess., 47(1): 89–108.
Poisson, C., Rouas, C., Manens, L., Dublineau, I. and Gueguen, Y. (2014a). Antioxidant status in rat kidneys after coexposure to uranium and gentamicin. Hum. Exp. Toxicol., 33(2): 136–147.
Poisson, C., Stefani, J., Manens, L., Delissen, O., Suhard, D., Tessier, C., Dublineau, I. and Guéguen, Y. (2014b). Chronic uranium exposure dose-dependently induces glutathione in rats without any nephrotoxicity. Free Radic. Res., 48(10): 1218–1231.
Polednak, A.P. and Frome, E.L. (1981). Mortality among men employed between 1943 and 1947 at a uranium-processing plant. J. Occup. Med., 23(3): 169–178.
Prat, O., Vercouter, T., Ansoborlo, E., Fichet, P., Perret, P., Kurttio, P. and Salonen, L. (2009). Uranium speciation in drinking water from drilled wells in Southern Finland and its potential links to health effects. Environ. Sci. Technol., 43: 3941–3946.
Prat, O., Bérenguer, F., Steinmetz, G., Ruat, S., Sage, N. and Quéméneur, E. (2010). Alterations in gene expression in cultured human cells after acute exposure to uranium salt: Involvement of a mineralization regulator. Toxicol. In Vitro, 24(1): 160–168.
Priest, N.D. (2001). Toxicity of depleted uranium. Lancet, 357(9252): 244–246.
Priest, N.D. and Thirlwall, M. (2001). Early results of studies on the levels of depleted uranium excreted by Balkan residents. Arch. Oncology, 9(4): 237–240.
Pujadas Bigi, M.M., Lemlich, P.M. and Ubios, A.M. (2003). Exposure to oral uranyl nitrate delays tooth eruption and development. Health Phys., 84(2): 163–169.
Pujadas Bigi, M.M. and Ubios, A.M. (2007). Catch-up of delayed tooth eruption associated with uranium intoxication. Health Phys., 92(4): 345–348.
Qi, L., Basset, C., Averseng, O., Quéméneur, E., Hagège, A. and Vidaud, C. (2014). Characterization of UO22+ binding to osteopontin, a highly phosphorylated protein: insights into potential mechanisms of uranyl accumulation in bones. Metabolomics, 6: 166–176.
Rached, E., Hoffmann, D., Blumbach, K., Weber, K., Dekant, W. and Mally, A. (2008). Evaluation of putative biomarkers of nephrotoxicity after exposure to ochratoxin in vivo and in vitro. Toxicol. Sci., 103(2): 371–381.
Radespiel-Tröger, M. and Meyer, M. (2013). Association between drinking water uranium content and cancer risk in Bavaria, Germany. Int. Arch. Occup. Environ. Health, 86: 767–776.
Radulescu, A., David, V.L., Trailescu, M., Iacob, R.E. and Boia, E.S. (2009). Reproductive toxicity induced by uranyl acetate dehydrate. Jurnalul Pediatrului, XII(47–48): 45–53.
Raff, O. and R.D. Wilken. (1999). Removal of dissolved uranium by nanofiltration. Desalination, 122: 147–150.
Rasmussen, P.E., Subramanian, K.S. and Jessiman, B.J. (2001). A multi-element profile of house dust in relation to exterior dust and soils in the city of Ottawa, Canada. Sci. Total Environ., 267(1–3): 125–140.
Ray, P.D., Huang, B. and Tsuji, Y. (2012). Reactive oxygen species (ROS) homeostasis and redox regulation in cellular signaling. Cell. Signalling, 24(5): 981–990.
Raymond-Whish, S., Mayer, L.P., O'Neal, T., Martinez, A., Sellers, M.A., Christian, P.J., Marion, S.L., Begay, C., Propper, C.R., Hoyer, P.B. and Dyer, C.A. (2007). Drinking water with uranium below the U.S. EPA water standard causes estrogen receptor-dependent responses in female mice. Environ. Health Perspect., 115(12): 1711–1716.
Rihs, S., Sturchio, N., Orlandini, K., Cheng, L., Teng, H., Fenter, P., and Bedzyk, M. (2004). Interaction of uranyl with calcite in the presence of EDTA. Environ. Sci. Technol., 38: 5078–5086.
Ritz, B., Morgenstern, H., Crawford-Brown, D. and Young, B. (2000). The effects of internal radiation exposure on cancer mortality in nuclear workers at Rocketdyne/Atomics International. Environ. Health Perspect., 108(8): 743–751.
Roach, J.D. and Zapien, J.H.. (2009). Inorganic ligand-modified, colloid-enhanced ultrafiltration: A novel method for removing uranium from aqueous solution. Water Res., 43: 4751–4759.
Rouas, C., Stefani, J., Grison, S., Grandcolas, L., Baudelin, C., Dublineau, I., Pallardy, M. and Gueguen, Y. (2011). Effect of nephrotoxic treatment with gentamicin on rats chronically exposed to uranium. Toxicology, 279(1–3): 27–35.
Saskatchewan Water Security Agency (2015). Personal communication with Ferris, S.
SCAHT (2015). Human and environmental impact of uranium derived from mineral phosphate fertilizers. SCAHT report for BLW. (Report no. SCAHT/NR/2015-06-19). Available at: www.blw.admin.ch/themen/00011/00076/index.html
SCC (2018). Directory of accredited product, process and service certification bodies. Standards Council of Canada, Ottawa, Ontario. Available at: www.scc.ca/en/accreditation/product-process-and-service-certification/directory-of-accreditedclients.
Schock, M.R. (2005). Distribution systems and reservoirs and reactors for inorganic contaminants. Chapter 6 in: MacPhee, M.J.(ed.) Distribution water quality challenges in the 21st century, 1st edition. Am. Water Works Assoc., Denver, Colorado.
Schock, M. and Lytle, D. (2011). Chapter 20: Internal corrosion and deposition control. In: Water quality and treatment: a handbook on drinking water. 6th edition. J.K. Edzwald (ed.), McGraw Hill and American Water Works Association, Denver, Colorado.
Scott, R. and Barker, F. (1969). Data on uranium and radium in groundwater in the United States 1954 to 1957. United States Department of the Interior, Washington, DC (Geological Survey professional paper 426).
Sengupta, P. (2013). The laboratory rat: Relating its age with humans. Int. J. Prev. Med., 4: 624–630.
Seiler, R.L. (2004). Temporal changes in water quality at a childhood leukemia cluster. Ground Water, 42(3): 446–455.
Seldén, A.I., Lundholm, C., Edlund, B. , Högdahl, C., Ek, B.M., Bergström, B.E. and Ohlson, C.G. (2009). Nephrotoxicity of uranium in drinking water from private drilled wells. Environ. Res., 109(4): 486–494.
Senko, O., Istok, J., Suflita, J., and Krumholz, L. (2002). In-situ evidence for uranium immobilization and remobilization. Environ., Sci. Technol. 36: 1491–1496.
Senko, O., Suflita, J., and Krumholz, L. (2005). Geochemical controls on microbial nitrate-dependent U(IV) oxidation. Geomicrobiology J., 22: 371–378.
Sheets, R.W. and Thompson, C.C. (1995). Accidental contamination from uranium compounds through contact with ceramic dinnerware. Sci. Total Environ., 175(1): 81–84.
Shetty, Y.R., Hedge, A.M. and Kar, A. (2016). Observation of teeth eruption timings among a group of children residing near uranium mines. Int. J. Oral Health Med. Res., 2(5): 9-12.
Shiraishi, K., Tagami, K., Muramastu, Y. and Yamamoto, M. (2000). Contributions of 18 food categories to intakes of 232Th and 238U in Japan. Health Phys., 78(1): 28–36.
Shuibo, X., Chun, Z., xinghuo, Z., Jing, Y., Xiaojian, Z., and Jingsong, W. (2009). Removal of uranium (VI) from aqueous solution by adsorption of hematite. J. of Env. Radioactivity 100: 162–166.
Shvartsbeyn, M., Tuchinda, P., Gaitens, J., Squibb, K.S., McDiarmid, M.A. and Gaspari, A.A. (2011). Patch testing with uranyl acetate in veterans exposed to depleted uranium during the 1991 Gulf War and the Iraqui Conflict. Dermatitis, 22(1): 33–39.
Sorg, T.J. (1988). Methods for removing uranium from drinking water. J. Am. Water Works Assoc., 80(7): 105–111.
Spalding, R.F. and Sackett, W.M. (1972). Uranium in runoff from the Gulf of Mexico Distributive Province: Anomalous concentrations. Science, 175(4022): 629–631.
Spencer, H., Osis, D., Fisenne, I.M., Perry, P.M. and Harley, N.H. (1990). Measured intake and excretion patterns of naturally occurring 234U, 238U, and calcium in humans. Radiat. Res., 124: 90–95.
Squibb, K.S. and McDiarmid, M.A. (2006). Depleted uranium exposure and health effects in Gulf War veterans. Phil. Trans. R. Soc. B, 361: 639–648.
Starosciak, E. and Rosiak, L. (2015). Determination of uranium reference levels in the urine of Warsaw residents (Poland). J. Radioanal. Nucl. Chem., 304: 75–79.
Stearns, D.M., Yazzie, M., Bradley, A.S., Coryell, V.H., Shelley, J.T., Ashby, A., Asplund, C.S. and Lantz, R.C. (2005). Uranyl acetate induces hprt mutations and uranium-DNA adducts in Chinese hamster ovary EM9 cells. Mutagenesis, 20(6): 417–423.
Stewart, B., Cismasu, A., Williams, K., Peyton, B., Peter S., Nico, P. (2015). Reactivity of uranium and ferrous iron with natural iron oxyhydroxides. Environ. Sci. Technol., 49: 10357–10366.
Straub, K.L., Benz, M. and Schink, B. (2001). Iron metabolism in anoxic environments at near neutral pH. FEMS Microbiol. Ecol., 34(3): 181-186.
Sullivan, M.F. (1980a). Absorption of actinide elements from the gastrointestinal tract of rats, guinea pigs and dogs. Health Phys., 38(Feb.): 159–171.
Sullivan, M.F. (1980b). Absorption of actinide elements from the gastrointestinal tract of neonatal animals. Health Phys., 38(Feb.): 173–185.
Sullivan, M.F., Ruemmler, P.S., Ryan, J.L. and Buschbom, R.L. (1986). Influence of oxidizing or reducing agents on gastrointestinal absorption of U, pu, am, cm, and pm by rats. Health Phys., 50(2): 223–232.
Tadmor, J. (1986). Atmospheric release of volatilized species of radioelements from coal-fired plants. Health Phys., 50(2): 270–273.
Tamburini, J.U. and Habenicht, W.L. (1992). Volunteers integral to small system's success. J. Am. Water Works Assoc., 84(5): 56–61.
Tannenbaum, A. and Silverstone, H. (1951). Some aspects of the toxicology of uranium compounds. In: Toxicology of uranium compounds. Tannenbaum, A. (ed.). McGraw-Hill, New York, NY, pp. 59–96.
Taulan, M., Paquet, F., Maubert, C., Delissen, O., Demaille, J., and Romey, M.C. (2004). Renal toxicogenomic response to chronic uranyl nitrate insult in mice. Environ. Health Perspect., 112(16): 1628–1635.
Tessier, C., Suhard, D., Rebière, F., Souidi, M., Dublineau, I. and Aragande, M. (2012). Uranium microdistribution in renal cortex of rats after chronic exposure: A study by secondary ion mass spectrometry microscopy. Micros. Microanal., 18: 123–133.
Thatcher, L.L., Janzer, V.J. and Edwards, K. (1977a). Methods for determination of radioactive substances in water and fluvial sediments - uranium, dissolved fluorometric method - direct (R-1180-76). In: Techniques of water-resources investigations of the United States Geological Survey. Washington, DC. pp. 83–88.
Thatcher, L.L., Janzer, V.J. and Edwards, K. (1977b). Methods for determination of radioactive substances in water and fluvial sediments - uranium, dissolved fluorometric method - extraction procedure (R-1181-76). In: Techniques of water-resources investigations of the United States Geological Survey. Washington, DC. pp. 89–92.
Thiébault, C., Carrière, M., Milgram, S., Simon, A., Avoscan, L. and Gouget, B. (2007). Uranium induces apoptosis and is genotoxic to normal rat kidney (NRK-52E) proximal cells. Toxicol. Sci., 98(2): 479–487.
Thomas, P.A. (2000). Radionuclides in the terrestrial ecosystem near a Canadian uranium mill - Part I: Distribution and doses. Health Phys., 78(6): 614–624.
Tissandié, E., Guéguen, Y., Lobaccaro, J.M.A., Paquet, F., Aigueperse, J. and Souidi, M. (2006). Effects of depleted uranium after short-term exposure on vitamin D metabolism in rat. Arch. Toxicol., 80: 473-480.
Tissandié, E., Guéguen, Y., Lobaccaro, J.M.A., Grandcolas, L., Voisin, P., Aigueperse, J., Gourmelon, P. and Souidi, M. (2007). In vivo effects of chronic contamination with depleted uranium on vitamin D3 metabolism in rat. Biochim. Biophys. Acta, 1770: 266-272.
Tolmachev, S.Y., Kuwabara, J. and Nogushi, H. (2006). Concentration and daily excretion of uranium in urine of Japanese. Health Phys., 91(2): 144–153.
Tracy, B.L. and Meyerhof, D.P. (1987). Uranium concentrations in air near a Canadian uranium refinery. Atmos. Environ., 21(1): 165–172.
Tracy, B.L., Quinn, J.M., Lahey, J., Gilman, A.P., Mancuso, K., Yagminas, A.P. and Villeneuve, D.C. (1992). Absorption and retention of uranium from drinking water by rats and rabbits. Health Phys., 62(1): 65–73.
Ubios, A.M., Guglielmotti, M.B., Steimetz, T. and Cabrini, R.L. (1991). Uranium inhibits bone formation in physiologic alveolar bone modeling and remodeling. Environ. Res., 54: 17–23.
Ubios, A.M., Piloni, M.J., Marzorati, M., and Cabrini, R.L. (1994-1995). Bone growth is impaired by uranium intoxication. Acta Odontol. Latinoam., 8(2): 3–8.
U.S. DOE (1997a). U-04-RC Uranium in biological and environmental materials. In: Chieco, N.A. (ed.), HASL-300 Volume II Rev. 0: The procedures manual of the environmental measurements laboratory, 28th edition. U.S. Department of Energy, New York, New York.
U.S. DOE (1997b). U-01-E Uranium in urine - fluorimetry. In: Chieco, N.A.(ed.), HASL-300 Volume II Rev. 0: The procedures manual of the environmental measurements laboratory, 28th edition. U.S. Department of Energy, New York, New York.
U.S. EPA (1991). Environmental Protection Agency, 40 CFR Parts 141, and 142. National Primary Drinking Water Regulations; Radionuclides; Proposed Rule; Federal Register, 58(136): 33050-33127. Washington, DC (570-Z-91-049).
U.S. EPA (1994). Method 200.8 rev. 5.4 determination of trace elements in waters and wastes by inductively coupled plasma - mass spectrometry. Environmental Monitoring Systems Laboratory, Office of Research and Development, Cincinnati, Ohio.
U.S. EPA (1996). Technology transfer handbook. Management of water treatment plants residual. Office of Research and Development. Cincinnati, Ohio, 45268.
U.S. EPA (2005). A regulator's guide to the management of radioactive residuals from drinking water treatment technologies. Office of Water (4606M). Washington, DC. (Report No. EPA 815/R-05/004). Available at; www.epa.gov/sites/production/files/2015-05/documents/816-r-05-004.pdf
U.S. EPA (2009). Analytical feasibility support document for the second six-year review of existing national primary drinking water regulations. Office of Water, Washington, DC. (Report No. EPA 815/B-09/003).
U.S. EPA. (2010). Drinking water treatability database. Office of Research and Development, U.S. Environmental Protection Agency, Cincinnati, Ohio. Available at: http://iaspub.epa.gov/tdb/pages/general/home.do
U.S. EPA (2012). Radionuclides in drinking water. Compliance options: Treatment technology descriptions. U.S. Environmental Protection Agency, Cincinnati, Ohio. Available at: http://cfpub.epa.gov/safewater/radionuclides/radionuclides.cfm
U.S. EPA (2014). Analytical methods approved for drinking water compliance monitoring of radionuclides. Office of Water, Washington, DC. Available at: http://nepis.epa.gov/Exe/ZyPDF.cgi/P100J79C.PDF?Dockey=P100J79C.PDF
U.S. EPA (2015). Radionuclide Rule presentations. U.S. Environmental Protection Agency, Cincinnati, Ohio. Available at: www.epa.gov/dwreginfo/radionuclide-rule-presentations
U.S.G.S. (2013). Crustal geophysics and geochemistry science center introduction to ICP-MS. U.S. Geological Survey, Denver, Colorado. Available at: http://crustal.usgs.gov/laboratories/icpms/intro.html.
Vacquier, B.(2008). Analyse de la mortalité dans la cohorte française des mineurs d'uranium. Doctoral thesis. L'université Paris XI. Available at: www.irsn.fr/.
van Netten, C. and Morley, D.R. (1982). Uptake of uranium and molybdenum from uranium rich soils by barley. Intern. J. Environ. Stud., 19: 43–45.
Vicente-Vicente, L., Quiros, Y., Pérez-Barriocanal, F., López-Novoa, J.M., Lóoez-Hernández, F.J. and Morales, A.I. (2010). Nephrotoxicity of uranium: Pathophysiological, diagnostic and therapeutic perspectives. Toxicol. Environ. Chem., 118(2): 324–347.
Wade-Gueye, N.M., Delissen, O., Gourmelon, P., Aigueperse, J., Dublineau, I. and Souidi, M. (2012). Chronic exposure to natural uranium via drinking water affects bone in growing rats. Biochim. Biophys. Acta, 1820: 1121–1127.
Wagner, S., Burch, J.B., Bottai, M., Puett, R., Porter, D., Bolick-Aldrich, S., Temples, T., Wilkerson, R.C., Vena, J.E. and Hébert, J.R. (2011). Groundwater uranium and cancer incidence in South Carolina. Cancer Causes Control, 22(1): 41–50.
Wang, L., Chen, A.S.C. and Lewis, G.M. (2010a). Arsenic and uranium removal from drinking water by adsorptive media. U.S. EPA demonstration project at Upper Bodfish in Lake Isabella, CA. Final performance evaluation report. Cincinnati, Ohio. (Report No EPA 600/R-10/165).
Wang, L., Chen, A.S.C. and Wang, A. (2010b). Arsenic removal from drinking water by ion exchange. U.S. EPA demonstration project at Fruitland, ID. Final performance evaluation report. Cincinnati, Ohio. (Report No: EPA 600/R-10/152).
Wappelhorst, O., Kuhn, I., Heidenreich, H. and Markert, B. (2002). Transfer of selected elements from food into human milk. Applied Nutritional Investigation, 18: 316–322.
Waxweiler, R.J., Roscoe, R.J. and Archer, V.E. (1981). Secondary sex ratio of first born offspring of US uranium miners. In: Birth defects in the Four Corners area. Transcript of meeting held February 27, 1981. Wiese, W. (ed.). University of New Mexico School of Medicine, Albuquerque, NM, pp. 37–50.
Waxweiler, R.J., Archer, V.E., Roscoe, R.J., Watanabe, A. and Thun, M.J. (1983). Mortality patterns among a retrospective cohort of uranium mill workers. In: Epidemiology Applied to Health Physics, Proceedings of the Sixteenth Midyear Topical Meeting of the Health Physics Society, Albuquerque, New Mexico, January 9-13, 1983; 428–435.
Wazne, M., Meng, X., Korfiztis, G.P. and Christodoulatos, C. (2006). Carbonate effects on hexavalent uranium removal from water by nanocrystalline titanium dioxide. J. Haz. Materials 136: 47–52.
Weber, K., Achenbach, L. and Coates, J. (2006). Microbes pumping iron: Anaerobic microbial iron oxidation and reduction. Nat. Rev. Microbiol., 4 (10): 752−764.
Werner, E., Roth, P., Wendler, I. and Schramel, P. (1998). Renal thorium and uranium excretion in non-exposed subjects: Influence of age and gender. In: Proceedings of the IRPA regional symposium on radiation protection in neighbouring countries of Central Europe. Sabol, J. (ed.). Prague, Czech Republic. Faculty of Nuclear Sciences and Physical Engineering, Czech,Technical University, pp. 325–327.
Westerhoff, .P.K., Benn, T.M., Chen, A.S.C., Wang, L. and Cumming, L.J. (2008). Assessing arsenic removal by metal (hydr)oxide adsorptive media using rapid small scale column tests. Cincinnati, Ohio. (Report No: EPA/600-R-08/051).
White, S. and Bondietti, E. (1983). Removing uranium by current municipal water treatment processes. J. Am. Water Works Assoc., 75(7): 374.
WHO (World Health Organization) (2011). Uranium in drinking-water: Background for development of WHO guidelines for drinking-water quality. World Health Organization, WHO/SDE/WSH/03.04/118/Rev/1, Geneva, Switzerland.
Wieland, E., Tits, J. and Bradybury, M. (2004). The potential effect of cementitious colloids on radionuclide mobilization in a repository for radioactive waste. Appl. Geochem., 19(1): 119.
Wiese, W.H. and Skipper, B.J. (1986). Survey of reproductive outcomes in uranium and potash mine workers: results of first analysis. Ann. Ame. Conf. Gov. Ind. Hyg., 14: 187–192.
Witmans, M.R., McDuffie, H.H., Karunanayake, C., Kerrich, R. and Pahwa, P. (2008). An exploratory study of chemical elements in drinking water and non-Hodgkin's lymphoma. Toxicol. Environ. Chem., 90(5–6): 1227–1247.
Wrenn, M.E., Durbin, P.W., Howard, B., Lipsztein, J., Rundo, J., Still, E.T. and Willis, D.L. (1985). Metabolism of ingested U and Ra. Health Phys., 48(5): 601–633.
Wrenn, M.E., Singh, N.P., Ruth, H. and Burleigh, D. (1988). Gastrointestinal absorption of soluble uranium from drinking water. NTIS PB88-201231; (Report No: EPA 600-D-88-078).
Wrenn, M.E., Singh, N.P. and Ruth, H. (1989). Gastrointestinal absorption of soluble uranium from drinking water by man. Radiat. Prot. Dosim., 26(1): 119–122.
Yarlagadda, S., Gude, V.G., Camacho, L.M., Pinappu, S. and Deng, S. (2011). Potable water recovery from As, U, and F contaminated ground waters by direct contact membrane distillation process. J. Haz. Materials, 192: 1388–1394.
Yuile, C.L. (1973). Animal experiments. In: Handbook of experimental pharmacology. Hodges, H.C. (ed.). Vol. 36. Uranium, Plutonium, Transplutonic Elements. Springer-Verlag, Berlin, pp. 165–195.
Yukon Environmental Health Services (2015). Personal communication with Brooks, P.
Yusan, S.D., and Akyil, S. (2008). Adsorption of uranium (VI) from aqueous solutions by akaganeite. J. Haz. Mater., 160: 388–395.
Zapecza, O. S. and Szabo, Z. (1986). Natural radioactivity in ground water - A review.. In: D. Moody, J. Carr, E. Chase, and R. Poulson (eds). National water summary-1986. Hydrological events and ground-water quality. United States Geological Syrvey. Water supply paper 2325.U.S. government printing office. . Washington. DC. pp. 50–57. Available at: https://pubs.usgs.gov/wsp/2325/report.pdf
Zhang A. and Clifford, D.A. (1994). Exhaustion and regeneration of resins for uranium removal. J. Am. Water Works Assoc., 86(4): 228–241.
Appendix A: List of acronyms
- AA
- activated alumina
- ALP
- alkaline phosphatase
- AM
- adsorptive media
- ANSI
- American National Standards Institute
- AST
- aspartate aminotransferase
- ATP
- adenosine triphosphate
- ATSDR
- Agency for Toxic Substances and Disease Registry
- BAT
- best available technology
- BMG
- β2-microglobulin
- BMI
- body mass index
- BV
- bed volume
- bw
- body weight
- CAS
- chemical abstracts service
- CBMN
- cytokinesis-block-micronucleus centromere
- CCME
- Canadian Council of Ministers of the Environment
- CHMS
- Canadian Health Measures Survey
- CP
- ceruloplasmin
- CYP3A
- cytochrome P450, family 3, subfamily A
- DMT1
- divalent metal transporter 1
- DOC
- dissolved organic carbon
- DOM
- dissolved organic matter
- DU
- depleted uranium
- EBCT
- empty bed contact time
- F
- first filial generation
- FISH
- fluorescence in situ hybridization
- GAC
- granular activated carbon
- GFR
- glomerular filtration rate
- GI
- gastrointestinal
- GGT
- γ-glutamyl transferase
- GOT
- glutamic oxaloacetic transaminase
- gpm
- gallons per minute
- GPT
- glutamic pyearuvic transaminase
- GPx
- glutathione peroxidase
- GR
- glutathione reductase
- GSH
- reduced glutathione
- GSSG
- oxidized glutathione
- GST
- glutathione-S-transferase
- HIX
- hybrid ion exchange
- IARC
- International Agency for Research on Cancer
- ICP-MS
- inductively coupled plasma mass spectrometry
- ICRP
- Internal Commission on Radiological Protection
- IFN-γ
- interferon gamma
- IX
- ion exchange
- HBV
- health-based value
Page details
- Date modified: