Guidelines for Canadian Recreational Water Quality: Understanding and Managing Risks in Recreational Waters
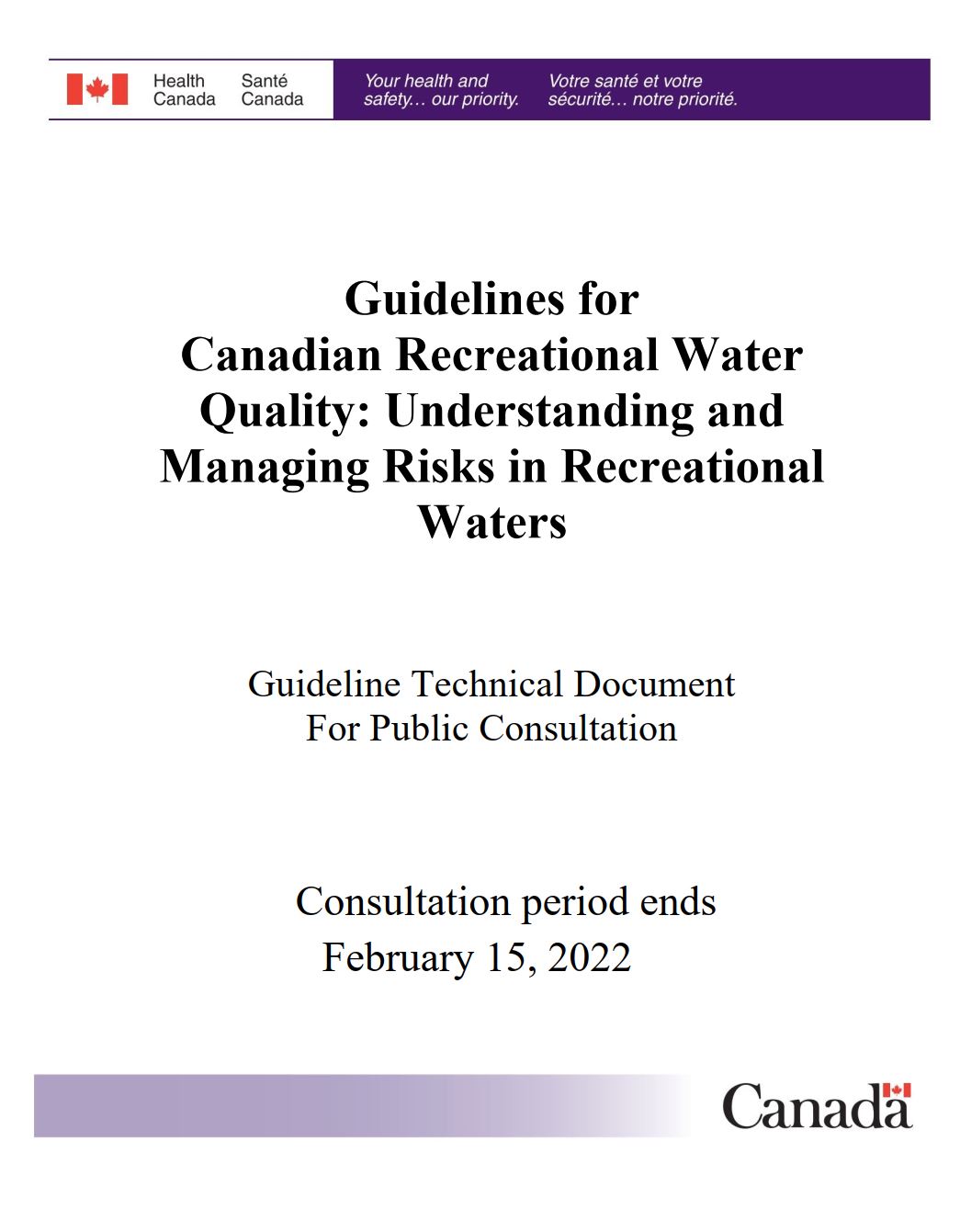
Download the alternative format
(PDF format, 1500 KB, 55 pages)
Organization: Health Canada
Type: PDF
Published: 2021-12-17
Purpose of consultation
This guideline technical document evaluated the available information on managing risks in recreational areas with the intent of updating/recommending a preventive risk management approach to managing recreational areas. The purpose of this consultation is to solicit comments on the proposed approach and on the potential impacts of implementing the recommendations.
The document was reviewed by external experts and subsequently revised. We now seek comments from the public. This document is available for a 60-day public consultation period. Please send comments (with rationale, where required) to Health Canada via email at water-eau@hc-sc.gc.ca.
All comments must be received before February 15, 2022. Comments received as part of this consultation will be shared with the recreational water quality working group members, along with the name and affiliation of their author. Authors who do not want their name and affiliation shared with recreational water quality working group members should provide a statement to this effect along with their comments.
It should be noted that this guideline technical document will be revised following the evaluation of comments received, and the recreational water quality guideline will be updated, if required. This document should be considered as a draft for comment only.
Table of contents
- 1.0 A Risk Management Approach
- 2.0 Environmental Health and Safety Survey
- 3.0 Water Quality Monitoring
- 4.0 Beach Sand Management
- 5.0 Beach Management Best Practices
- 6.0 Public Awareness and Communication
- 7.0 Public Health Advice
- 8.0 References
- Appendix A: List of abbreviations
- Appendix B: Recreational swimming area environmental health and safety survey (EHSS) checklist
- Appendix C: Informative beach signs
Foreword
The Guidelines for Canadian Recreational Water Quality are comprised of multiple guideline technical documents that consider the various factors that could interfere with the safety of recreational waters from a human health perspective. They provide guideline values for specific parameters used to monitor water quality hazards, and recommend science-based monitoring and risk management strategies. Recreational waters are considered to be any natural fresh, marine or estuarine bodies of water that are used for recreational purposes; this includes lakes, rivers, and human-made constructions (e.g., quarries, artificial lakes) that are filled with untreated natural waters. Jurisdictions may choose to apply these guidelines to other natural waters to which limited treatment is applied (e.g., short-term use of disinfection for an athletic event). However, in such situations, caution should be exercised in applying the guidelines as indicator organisms are easier to disinfect then other disease-causing microorganisms (e.g., protozoan pathogens). Recreational activities that could present a human health risk through intentional or incidental immersion and ingestion include primary contact activities (e.g., swimming, bathing, wading, windsurfing and waterskiing) and secondary contact activities (e.g., canoeing and fishing).
Each guideline technical document has been established on the basis of current, published scientific research related to health effects, aesthetic effects, and beach management considerations. Recreational water quality generally falls under provincial and territorial jurisdiction and therefore the policies and approaches will vary between jurisdictions. The guideline technical documents are intended to guide decisions by provincial and local authorities that are responsible for the management of recreational waters. For a complete list of the guideline technical documents, please refer to the Guidelines for Canadian Recreational Water Quality summary document on the Health Canada website.
Understanding and Managing Risks in Recreational Waters
The authority charged with the day-to-day oversight of the recreational water area generally has the most comprehensive knowledge of the area and is therefore in the best position to take the actions necessary to ensure safe water recreation. The management information in this section is more pertinent to managed beaches (either public or private); however, the same principles can be applied to any natural water area that has been designated as a recreational area. Effective recreational water management requires the cooperation of all stakeholders, including beach operators and service providers, governments, local businesses and industry, as well as users. All stakeholders are expected to become informed about their roles and responsibilities in the management of recreational waters.
The best strategy for protecting public health from risks associated with recreational waters is a preventive risk management approach that focuses on the identification and control of water quality hazards and their associated risks, combined with microbial water quality assessments. Reactive management strategies that rely on microbial water quality monitoring alone are not sufficient to protect the health of recreational water users.
1.0 A Risk Management Approach
A preventive risk management approach that incorporates procedures, actions and tools to collectively reduce the risk of human exposure to recreational water quality hazards is recognized by water quality professionals worldwide as the preferred approach to protecting public health. This approach is based on concepts that mirror those found in drinking water frameworks, such as the source-to-tap approach (CCME, 2004), and in drinking water management plans, such as water safety plans (WHO, 2004). Engaging in recreational water activities, regardless of whether it is swimming, splashing, or other activities, will always involve some level of associated risk. The goal of a risk management approach is to make decisions that are based on an assessment of the possible health risks to recreational water users balanced against the significant benefits that recreational water activities provide in terms of health and enjoyment. The concepts of preventive risk management were at the heart of the recommendations for improved management of recreational waters proposed by an international panel of experts and published in the document that has come to be known as the "Annapolis Protocol" (WHO, 1999). Similarly, it is this approach that formed the basis for the management framework outlined in the Guidelines for Safe Recreational Water Environments (WHO, 2003).
A preventive risk management approach succeeds by incorporating multiple areas of management (e.g., source protection, hazard assessment and prioritization, monitoring, hazard control, communication, consultation, and training), rather than focusing all efforts in a single area. Specific benefits include:
- more effective public health protection;
- improved recreational water management, i.e., operational plans can be specifically tailored to address an area's individual needs and resources;
- improved public communication on how individuals can protect themselves while enjoying recreational waters (e.g., not swallowing water), and the public's role in ensuring recreational water safety;
- increased public confidence in the recreational water quality; and
- better management of emergencies, i.e., water quality hazards are understood and plans are in place to address the problems effectively.
A key component of a preventive approach is situation assessments using an environmental health and safety survey (EHSS) (section 2). Other components include water quality monitoring (section 3), beach sand management (section 4), beach management best practices (section 5), public awareness and communication (section 6), and public health advice (section 7).
2.0 Environmental Health and Safety Survey
An environmental health and safety survey (EHSS) provides the foundation or "blueprint" for designing and implementing an effective risk management plan for recreational waters. It is a comprehensive search for, and assessment of, existing and potential water quality hazards (biological, chemical and physical) and their associated risks to the health and safety of the public at designated beach areas. The EHSS also represents a general review of all aspects of a beach's operation. Similar approaches, such as beach sanitary surveys or sanitary inspections, are used in other jurisdictions (NHMRC, 2008; US EPA, 2008; US EPA, 2013; Government of Alberta, 2019). The data collected during an EHSS provide beach operators, service providers and responsible authorities with the information necessary to make sound risk management decisions and to develop and maintain an effective beach monitoring program. The EHSS process consists of three basic steps: pre-survey preparations, the on-site visit and the assessment report.
An EHSS should be conducted on an annual basis, just before the start of the swimming season. This survey should:
- catalogue the recreational water area's basic characteristics;
- identify any potential sources of fecal contamination in the immediate recreational area as well sources that may be impacting the area from the larger watershed;
- identify any other potential physical, chemical or biological water quality hazards that may present a risk to recreational water users; and
- evaluate the effectiveness of the monitoring programs and risk management measures currently in place.
The authority with the most knowledge of the day-to-day operation of the beach is the best candidate to lead this process. The EHSS process can also benefit greatly from intersectoral collaboration as not all potential impacts may be known to the candidate that is the lead. Persons or groups who may have valuable insight and information to support the process can include:
- the appropriate provincial or territorial management or regulatory authority;
- beach managers;
- public and environmental health departments;
- wastewater infrastructure managers;
- local universities and water researchers;
- community members;
- watershed or lake management groups; and
- individuals representing local business and industry.
2.1 Pre-survey preparations
The pre-survey preparation step involves the collection and review of all information available on the beach and adjacent area, including reports of any previous surveys. It can provide valuable information on historical trends, problems and successes, which will help ensure a more thorough and efficient on-site visit. The type and quantity of information on any given beach will vary, but it is important to gather whatever information is available. Initial preparations may begin with a review of basic beach information, such as the beach's physical characteristics, the types of activities practised and estimates of beach attendance. The use of topographical maps, aerial photos and geographic information system (GIS) data (including delineation of any sanitary and stormwater infrastructure) can provide additional perspective and help in the identification of contamination sources, potential sampling sites and nearby land uses. The examination of historically accumulated data relating to microbiological results, cyanobacterial blooms, beach postings, and disease surveillance will provide information on the area's suitability for recreation and the potential risks to swimmers. Assessment of hydrological, meteorological and other information on rainfall, currents, tides, and prevailing winds can help identify their impact (either singly or collectively) on water quality. Information on potential waste discharges (e.g., sewage, storm drains) and, in wet weather, sources of non-point runoff, should also be collected. Regular communication between beach managers and agencies with wastewater infrastructure responsibilities can be important for identifying changes to sanitary or stormwater systems that could have impacts on recreational water areas.
2.2 On-site visit
The purpose of the on-site visit is to visually identify and confirm any existing or potential water quality hazards. Information may be collected on the existence and adequacy of public facilities, safety provisions, and signage used for public awareness and communication. For the purposes of this EHSS, a hazard is an object or condition that may increase human health risk or pose a safety issue. For most swimming areas, contact with fecal pollution in the environment is a significant concern; thus, attention should be paid to the potential sources of fecal contamination, both point sources (discharge or drainage that may contain sewage, stormwater or other fecal wastes) and diffuse sources (e.g., domestic and wild animals and birds, upstream livestock and crop operations, stormwater runoff from the beach and surrounding areas, septic wastes, contamination from swimmers themselves). The risk to human health is more significant and the relationship between the indicators of fecal contamination (E. coli or enterococci concentrations) and impacts on human health is more reliable when the contamination sources have the potential for high concentrations of human pathogens (e.g., human and ruminant feces). Identifying these fecal sources is essential for understanding beach water quality. For beaches where information on contamination sources is missing or incomplete, fecal pollution source tracking research can be considered (see Section 3.2). Alternatively, beach managers should take a conservative approach and assume that all fecal pollution is from high-risk human and ruminant fecal sources.
Additional hazards can include:
- chemical hazards (e.g., industrial discharges, contamination from marinas/watercraft);
- biological hazards (e.g., cyanobacterial blooms, organisms responsible for swimmer's itch); and
- physical hazards (e.g., litter, poor visibility, submerged items, riptides and strong currents).
Other information collected may be useful in identifying hazards that are less apparent. For example, the presence of large amounts of floating debris may be indicative of sewage (e.g., condoms, tampon applicators) or stormwater discharges. An example of an EHSS checklist, which includes the type of information to be collected during an on-site visit, is provided in Appendix B.
Additionally, it is advisable to conduct site visits under both dry and wet weather conditions and, in the case of marine waters under various tidal conditions. Certain contamination sources or events (e.g., runoff, stormwater discharges) may be visible only during rainfall periods, and discharge pipes may be visible only at low tides or dry periods. Representative water samples may also be collected and analyzed to confirm the presence of contamination and determine its variability and source (see Section 3.0). Shortened surveys may also be carried out throughout the swimming season at the time of microbiological monitoring; these can be used to collect more timely information about the recreational water area that can be helpful when interpreting the monitoring results. Such information has also been valuable in developing models aimed at predicting water quality (see Section 3.4).
2.3 Assessment report
Once the on-site visit is completed, priority water quality hazards need to be identified. Priorities should be based on the likelihood of exposure to a given hazard and the associated consequence. Factors that may contribute to a swimmer's exposure also need to be considered. These may include the proximity of the hazard to the swimming area, effects of the area's physical characteristics (depth, water circulation), potential weather influences, types and patterns of recreational activities practised in the area and impacts of any existing barriers. Particular attention should be directed towards identification and assessment of sources of human sewage and cattle fecal wastes. For example, in the case of a sewage cross-connection into a stormwater outfall or a combined sewer overflow, factors contributing to swimmer exposure include heavy rains causing a discharge of sewage material, currents or winds driving this material towards the swimming area and the absence of public communication methods to alert the public that contact with the water should be avoided for a period immediately following heavy rainfall. In some instances, the information collected may be used to help inform a quantitative assessment of potential health risks (see Section 3.3). The risk assessment may also be used to identify potential points at which additional barriers may be needed to reduce the degree of human exposure.
The process should culminate in an assessment report which should be used when developing further beach management or operational plans. In addition to reporting on the pre-survey preparations and the survey findings, it should specify priorities for action, identify barriers that may be implemented to protect recreational users and provide recommendations for an appropriate beach monitoring program, which should include water quality sampling as well as routine actions (e.g., shortened beach surveys) that monitor the implemented barriers identified in the EHSS. The flowchart in Figure 1 (modified from Codd et al., 2005) shows a possible sequence of events when designing and implementing a plan to manage the risks for recreational waters. It may be used as a guide for beach operators, service providers or responsible authorities wishing to develop their own operational plans.
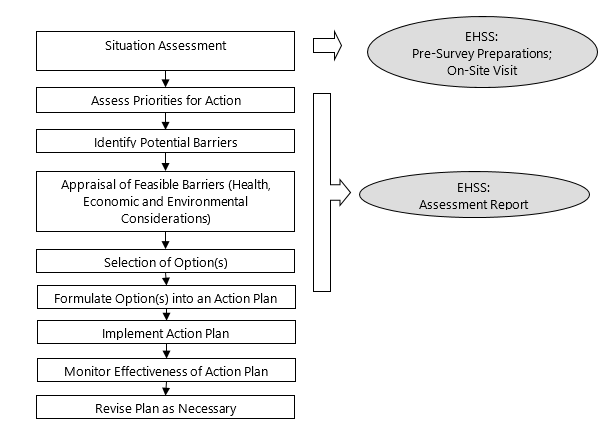
Figure 1. Sequence of events for designing and implementing a risk management strategy for recreational waters.
This figure shows a possible sequence of events when designing and implementing a plan to manage the risks for recreational waters. This sequence begins with a situation assessment and continues to an assessment of priorities for action, the identifying of potential barriers, the appraisal of feasible barriers, a selection of options, the formulation of options into an action plan, the implementation of an action plan, the monitoring of the action plan's effectiveness, and the revision of the plan as necessary.
3.0 Water Quality Monitoring
Water quality monitoring at recreational beaches should be included as part of a risk management strategy and can serve many functions. It can be used to:
- compare water quality to guideline values;
- identify the impacts of water quality events;
- demonstrate long-term water quality trends;
- support EHSS findings or identify gaps;
- verify that mitigation strategies (e.g., notifications, corrective actions) are put in place; and
- verify that these strategies are operating effectively.
Water quality monitoring may be conducted routinely or may be carried out for specific hazards (e.g., during a suspected outbreak or in response to an unusual wastewater discharge) when there is evidence of water quality issues or when further information is needed.
Routine monitoring usually includes indicators that inform beach managers about the potential impacts of fecal contamination, such as the fecal indicators E. coli or enterococci (see Section 3.1) as well as other types of parameters (i.e., cyanobacterial indicators or toxins, aesthetic quality, physical hazards, specific hazards) (see Sections 3.5 and 3.6).
Targeted water quality monitoring for specific hazards may be necessary in the following situations:
- identifying fecal pollution sources to inform decision making on level of risk associated with a recreational area (see Sections 3.2 and 3.3);
- reports of a disease outbreak or illnesses of specific etiology;
- reports of a suspected illness of undetermined cause;
- reports of water-related injuries;
- levels of an indicator strongly suggesting the presence of a specific hazard;
- reports of a specific event such as a sewage or chemical spill; or
- visual reports of the development of a cyanobacteria bloom.
Routine and targeted monitoring data can also be used in setting up predictive models for beach areas (see Section 3.4).
3.1 Monitoring for fecal indicators
Recreational waters should be routinely monitored to characterize the level of fecal pollution. The primary indicators used are E. coli or enterococci. Risk-based thresholds have been developed for these indicators to protect public health. Other fecal indicators may provide valuable supplemental information with respect to the fecal contamination of recreational waters. They may be included in a monitoring program or for fecal source tracking studies. A notable area of current research is the development of water quality risk-based thresholds for some of the other fecal indicators (e.g., coliphages, HF183 and crAssphage microbial source tracking marker genes for human sewage). Further information can be found in Section 3.2 and in Health Canada (in publication-a).
A well-structured and planned monitoring program is essential for assessing and communicating information on the quality of recreational waters. There are a number of factors that can influence the spatial and temporal microbiological quality of a recreational water body. For example, studies have reported higher concentrations of indicator bacteria in samples collected in the mornings, with concentrations decreasing later in the day (US EPA, 2005a; US EPA, 2010). In addition to the time of day, the type and periodicity of contamination events (both point and non-point sources), recent weather conditions, the number of users frequenting the swimming area and the physical characteristics of the area itself can impact water quality. Consequently, significant day-to-day (and within-day) variation in indicator organism densities has been well documented for recreational waters (Leecaster and Weisberg, 2001; Boehm et al., 2002; Whitman and Nevers, 2004; US EPA, 2005a). As a result of this variation, it is important to have a well-designed monitoring program and to understand how the information obtained can be best used to inform public health decisions. Monitoring using PCR-based methods has the advantage of providing more rapid results for decision-making purposes, sometimes within a few hours of the time of analysis. Development of predictive beach water quality models may also be beneficial where water quality is variable and sufficient data are available. All monitoring data collected, whether daily or less frequently, provide information that allow the responsible authorities to more easily observe water quality trends and to make more informed decisions regarding the area's overall suitability for recreation.
Decisions regarding the frequency of monitoring, the areas to be monitored, choice of indicators and methods, and monitoring program design (including the number of samples and the locations where they should be collected) will be made by the appropriate regulatory and management authorities. The monitoring program should capture the spatial variability of the beach area and incorporate information derived from the EHSS, taking into account recommendations made about priority areas of concern.
There should be a documented monitoring plan for all monitored beaches, providing, at a minimum, instructions on:
- the parameters to be analyzed;
- the locations (including GIS coordinates) at which samples are to be collected; and
- the times, sample collection depth and frequencies of sample collection.
In general, waters regularly used for primary contact recreational activities should be monitored for fecal indicators at a minimum frequency of once per week during the swimming season. A weekly monitoring strategy allows comparison of the water quality results to the guideline values. It is also useful to alert managers and responsible authorities to more persistent contamination problems that may have developed and allows them to make the necessary decisions within a reasonable time frame. More frequent monitoring should be considered for high-use beaches. These recommendations are consistent with those published by the United States Environmental Protection Agency (US EPA) in its 2012 Recreational Water Quality Criteria (US EPA, 2012).
Beaches may also have classification systems that consider a number of factors in assigning an overall rating to the beach. For such systems, it is suggested that a minimum of 60 samples should be used to characterize water quality, although a greater number of samples (e.g., closer to 100) provides a more precise estimate (WHO, 2009). As many beaches do not collect this number of samples yearly, water quality results from multiple bathing seasons (when no substantive change has occurred in the watershed) can be combined.
When developing sampling programs, consideration should also be given to collecting samples to characterize event-driven episodes of pollution that may affect recreational waters—for example, immediately following periods of heavy rainfall or at times of greatest swimmer activity. Additional factors that may be considered during the design and implementation of recreational water monitoring programs are described in more detail elsewhere (Bartram and Rees, 2000; US EPA, 2010).
Less frequent monitoring may be possible under certain circumstances, such as at beaches in remote locations or in areas where primary contact recreational activities are not a regular occurrence. Beaches that have historically demonstrated water quality results well below the guideline values through relatively intensive monitoring and the use of an EHSS may also be able to reduce their sampling frequency to help ease the burden of monitoring (Bartram and Rees, 2000; WHO, 2003). Thus, if it can be determined that a recreational water area is of consistently good microbiological quality, does not have any obvious sources of fecal contamination and is not considered to present a significant risk to the health and safety of its users, monitoring may be reduced to a frequency that is sufficient to verify that the conditions have not deteriorated. It may also be acceptable to reduce monitoring frequencies for recreational water areas that consistently demonstrate poor water quality results, but only where appropriate management actions are taken to discourage recreational use and the risks are clearly communicated to the public.
3.1.1 Location of microbiological sampling
Most bodies of water used for recreational purposes are not completely homogeneous with respect to their microbiological properties. In recreational water evaluations, the purpose of sampling is to obtain aliquots that are as representative as possible of microbiological quality of the area. A single water sample provides a quantitative estimate of the indicator bacteria present at a particular site and time. Multiple samples may be needed to provide a more accurate estimate of the water quality at the time of sampling (Whitman and Nevers, 2004; US EPA, 2005a; US EPA, 2010). The number of sampling locations included during a sampling event should be based on the beach characteristics as well as the fecal sources identified as part of the EHSS. However, as the total number of samples increases, the more representative the data will be of the overall water quality.
Sampling sites should be chosen to be representative of the water quality encountered throughout the entire swimming area. This should include points of greatest swimmer activity, as well as peripheral points subject to external fecal pollution. Stormwater, sewage or river outlets can give certain sections of a body of water microbiological qualities that are very different from those of the water body at large. If there are distinct areas that are highly influenced by a pollution source and they can be delineated from the rest of the beach area, it is possible to sample these areas separately. At some longer beaches, sources of fecal pollution can vary significantly along the length of the beach (Edge et al., 2010). The degree of heterogeneity in a water area can also be affected by rainfall, wind direction and velocity, currents and tides, or the presence of physical barriers, such as sandbars, natural or artificial wave breaks and piers.
Further guidance on developing sampling plans has been published elsewhere (US EPA, 2010).
The depth at which samples are collected can have a significant effect on the resulting estimates of water qualityFootnote 1. Where the water is very shallow, disturbances of the foreshore sand and sediment caused by wave swash and swimmer activity can result in the resuspension of fecal indicator microorganisms (Whitman and Nevers, 2003; Vogel et al., 2016; Palmer et al., 2020). This resuspension may inflate microbiological estimates but not necessarily reflect an increase in bather risk if pathogens are not also present in the sediments. Where the water is deeper, this effect has less influence on water quality measurements. In contrast, deeper waters are relatively more exposed to offshore fecal sources than shallower waters (US EPA, 2005a). Adult chest depth (approximately 1.2 to 1.5 m from the bottom) has historically been the most common sampling depth, although newer epidemiological studies have also used waist depth (approximately 1 m). Traditionally, this has been considered to represent the depth of greatest swimmer activity and the location nearest to the point of head immersion, which would be indicative of the risk associated with accidentally swallowing water. However, sampling at shallower depths (ankle or knee depth—approximately 0.15 to 0.5 m from the bottom) may be more representative of water quality encountered by young children playing at the water's edge. Epidemiological studies have typically found that only samples collected at depths greater then knee depth show a mathematical relationship between indicator organism density and swimmer illnesses (US EPA, 2010). It is suggested that a reasonable but still conservative approach to monitoring may be to sample at waist depth (US EPA, 2010). The sample depth (or depths) selected for an individual beach should be determined by the local or regional authority in order to obtain the best information for their particular recreational water area. However, if more than one sampling depth is included in the monitoring plan, all samples collected at a specific depth should be analyzed as a single group (e.g., for calculating the geometric mean) in order to improve the precision of the data.
3.1.2 Composite sampling
Composite sampling provides a way to increase the area covered under a beach monitoring program, while potentially minimizing the costs associated with analysis. Composite sampling involves the collection of multiple samples from across a stretch of beach, combining them into one large composite, and then analyzing a subsample of the resulting mixture. There are some challenges with the use of this technique. Increased sampling is required initially to validate whether composite sampling will be feasible at a given area. The area must be characterized to identify hot spots (sampling points having continuously poor water quality) that can disrupt the analysis. Some level of statistical knowledge is required to analyze the data. Nevertheless, investigations have suggested that, if properly conducted, composite sampling can be used to make water quality decisions with a degree of accuracy comparable to that achieved by analyzing the samples individually and averaging the results (Kinzelman et al., 2006; Bertke, 2007; Reicherts and Emerson, 2010). Further information on composite sampling can be found in the Guidelines for Canadian Recreational Water Quality – Microbiological Methods technical document (Health Canada, in publication-b.
3.2 Fecal pollution source tracking methods
Fecal source tracking can be used to supplement the monitoring and assessment tools that are already in place at recreational areas. Such tools should be designed to answer a specific question and should be based on the analysis of the available data, including bacteriological sampling, EHSS results, and local knowledge. In order to conduct source tracking, it is important to have a good understanding of the nature of the fecal pollution problem. The EHSS is a particularly useful tool for helping recreational water area operators, service providers and local authorities identify the potential sources of fecal material that are relevant to their recreational water area.
If fecal source tracking is being considered, there is a toolbox of methods available. A number of methods have been successfully used in recreational water settings to identify unexpected fecal pollution sources, to verify information from other lines of evidence, to resolve local beach closure problems involving limited fecal sources, and to break down large source tracking problems into more manageable studies (Kinzelman and McLellan, 2009; Converse et al., 2012; Edge et al., 2018; Edge et al., 2021). The choice of methods will depend on the question that needs to be answered, the suspected fecal sources, and the expertise of the researchers involved. The methods selected may differ depending on whether the study only needs to differentiate human from non-human sources, or whether the fecal pollution attribution needs to be broken down into more categories (i.e., human, cattle, wildlife, birds, etc.).
Fecal source tracking in recreational water areas often focuses on identifying sources with known human-pathogen contributing potential (i.e., human and ruminant feces), as even minor contributions from human sewage (e.g., 10% to 20% of the E. coli concentration) is sufficient to drive bather risk (Schoen and Ashbolt, 2010). Other animal markers may aid in clarifying observed or expected fecal sources but their relationship to potential disease impacts has not been clearly established (Schoen and Ashbolt, 2010). It should be noted that source tracking methods may not be able to identify all of the sources contributing fecal material to the watershed and the recreational water area. Also, although markers are considered host-specific, studies have reported some cross-reactivity with species other than the intended target, generally in fewer samples and at lower concentrations than found in the target species (Nguyen et al., 2018; Staley et al., 2018b).
When designing a fecal source tracking study, a tiered approach should be considered. A tiered approach utilizes the currently available information on the watershed and recreational area (e.g., fecal indicator bacteria data, land use information, sanitary infrastructure conditions) to help determine fecal sources before instituting more complex chemical and microbiological source tracking methods. Any fecal source tracking assessment should also use a toolbox approach based on multiple lines of evidence to identify fecal pollution sources. Additional information on the tiered approach is available elsewhere (Griffith et al., 2013). Further guidance on designing source tracking studies can be found in publications from the US EPA (2005b; 2019), the Southern California Coastal Water Research Project (Griffith et al., 2013) and the US Geological Survey (Stoeckel, 2005). Source tracking is also included in the Alberta Safe Beach Protocol (Government of Alberta, 2019).
If a recreational area needs to use chemical and microbiological methods, a variety of methods are available. Understanding the fecal sources can help to assess potential public health risks, as sources of human and ruminant fecal contamination have been shown to be of greater concern than other animal sources (see Section 3.3). It can also help target appropriate risk management barriers, which in turn can reduce beach postings and prevent potential waterborne disease outbreaks.
3.2.1 Chemical methods
Chemical methods of analysis are based on the detection of chemical compounds known to be present in fecal material as a result of human activities—either through consumption and/or metabolism and the subsequent excretion in feces or via disposal as sewage wastes. Numerous chemical compounds have been investigated as potential markers for human sources of fecal pollution. Artificial sweeteners, caffeine, detergents, fluorescent whitening agents, fragrance materials, fecal sterols, fecal stanols, pharmaceuticals and personal care products have all been proposed as markers of fecal pollution from sewage treatment plants (Glassmeyer et al., 2005; Tran et al., 2015; Devane et al., 2019). Chemical tracers such as dyes have been used to confirm suspected point sources of contamination, such as wastewater outfalls. Some advantages to using chemical markers include a shorter analysis time than for many microbial methods, low detection limits, and ease of analysis (Haack et al., 2009). Potential drawbacks may include differing fates in the environment for chemical markers compared to microorganisms (Glassmeyer et al., 2005), lack of any consistent relationship with illness for risk modelling (Napier et al., 2018), non-fecal sources of some chemical markers (Tran et al., 2015), and costs. However, chemical markers can be used as part of multiple lines of evidence and a toolbox approach to understanding fecal sources.
3.2.2 Microbial methods
Microbial source tracking (MST) methods are based on the premise that certain fecal microorganisms are strongly associated with specific hosts and that particular attributes of these host-associated microorganisms can be used to determine fecal sources (Harwood et al., 2014). MST methods can be divided into library-dependent and library-independent methods. Library-dependent methods were more widely used in early microbial source tracking studies, whereas in recent investigations, library-independent methods are used almost exclusively.
Library-dependent methods establish a reference library of characteristics of individual fecal indicator bacteria isolates obtained from known fecal pollution sources and then compare these characteristics to those from "unknown" water sample isolates. For example, a library could be a database of antibiotic resistance profiles or DNA fingerprints of E. coli isolates obtained from animal feces and municipal wastewater effluent (Wiggins, 1996; Dombek et al., 2000; Carson et al., 2001; Edge and Hill, 2007). The similarity of the profiles or fingerprints of E. coli isolates obtained from recreational waters ("unknowns") can then be compared with the profiles or fingerprints in the library ("knowns") to make statistical inferences about the source of the waterborne E. coli isolates. There are numerous drawbacks to this type of method. They require large reference libraries for comparison to unknowns (smaller libraries with fewer than 1 000 biotypes tend to mislead in associations), are prone to misclassification of fecal sources (especially when the library size is limited), and the libraries are not transferable between locations (Griffith et al., 2003; Stoeckel et al., 2004). They are also labour intensive and can have long wait times for results. These drawbacks have generally led to a discontinuation of library-dependent methods that are based on collecting isolates for fecal indicator bacteria. Recently, however, there has been some investigation into using next-generation sequencing results for MST, which is a library-dependent method comparing DNA sequences from water samples to reference databases of DNA sequences from known host species (Staley et al., 2018a; Unno et al., 2018). The utility of this method still needs to be proven.
Library-independent methods are now more widely used. They involve detecting host-specific DNA markers or host-specific organisms (bacteria or viruses) to identify the sources of fecal contamination in the water. Most library-independent methods rely on PCR methods (qPCR, digital PCR, or, in early studies, end-point PCR) to detect the markers or organisms of interest. Examples of host-specific markers include toxin genes (Khatib et al., 2002, 2003), genes for virulence factors (Scott et al., 2005) and highly conserved DNA sequences (Bernhard and Field, 2000a; Johnston et al., 2010). In particular, human-specific markers within the 16S rDNA of the genus Bacteroides are widely used (Bernhard and Field, 2000b; Layton et al., 2006; Kildare et al., 2007; Okabe et al., 2007; Ahmed et al., 2009; Shanks et al., 2009; Green et al., 2014; Mayer et al., 2018). Animal host-specific DNA markers have also been developed, including avian, ruminant, and various pet markers (Bernhard and Fields, 2000a; Kildare et al., 2007; Lu et al., 2008; Shanks et al., 2008; Weidhaas et al., 2010; Green et al., 2012). The host-specific viruses most commonly researched are human adenovirus, human polyomaviruses, pepper mild mottle viruses, and the phage crAssphage (McQuaig et al., 2009; Ahmed et al., 2010; Wong et al., 2012; Rusiñol et al., 2014; Symonds et al., 2016; Farkas et al., 2019). Although there can be some geographic variability in host-specific markers and host-specific organisms, these methods have been applied successfully to MST studies in recreational waters worldwide (Boehm et al., 2003; Bower et al., 2005; Noble et al., 2006; Hughes et al., 2017; Cao et al., 2018; Nguyen et al., 2018; Staley et al., 2018b).
There are advantages and drawbacks to library-independent methods. On the positive side, these methods are less labour intensive than library-dependent methods and therefore are easier and less expensive to conduct. Some of the best host-specific markers (e.g., HF183 for human sources) have been shown to be transferrable across regions (Mayer et al., 2018) and may be related to bather illness (Boehm et al., 2015; Cao et al., 2018; Boehm et al., 2018; Boehm and Soller, 2020), although this relationship has not been consistently reported (Napier et al., 2017). Some human-specific markers (e.g., crAssphage) also occur at high concentrations in raw sewage (Farkas et al., 2019; Korajkic et al., 2020) and decay more slowly than other genetic markers (Ahmed et al., 2019), which makes them useful for fecal source tracking.
3.3 Quantitative microbial risk assessment
Quantitative microbial risk assessment (QMRA) is used to estimate the potential health risks associated with a specific exposure scenario. Assessments use water quality information, assumptions on exposure conditions, and dose-response models for specific pathogens as inputs to provide risk estimates. In recreational settings, QMRA has been used to better understand the relative potential health impacts from human pathogens, as well as to support decisions about health risk in water quality management plans.
3.3.1 Estimating health risks at beach sites
QMRA has been used in research studies to investigate the estimated risks from various pathogens at beach sites. The pathogens most often included are enteric viruses (e.g., noroviruses, human adenoviruses), enteric protozoa (e.g., Cryptosporidium, Giardia), and enteric bacteria (e.g., Campylobacter, Salmonella). QMRA studies are often conducted as an alternative to epidemiological studies, as the latter are expensive and labour intensive. QMRA studies can also predict risks to lower levels than can be identified using epidemiological studies. Some researchers have conducted QMRA studies in parallel with epidemiological studies to compare the health risk outcomes. For example, in a study investigating wet weather impacts on surfer health, similar gastrointestinal (GI) impacts were estimated when comparing the QMRA analysis to the epidemiological study results (15 GI per 1 000 recreation events vs 12 excess GI per 1 000 recreational events, respectively) (Soller et al., 2017). Another paired QMRA and epidemiological study investigating secondary contact activities reported approximately an order of magnitude difference in the health risk from the two approaches (Rijal et al., 2011; Dorevitch et al., 2012). QMRA methods have also been used to reanalyze epidemiological study data where no links to human health could be identified. In one study, the QMRA predicted 2 to 3 illnesses per 1 000 recreators, well below the level that would be detectable by the epidemiological study (Soller et al., 2016). These studies highlight the usefulness of QMRA for estimating human health risks in a recreational water quality setting.
3.3.2 Comparing health risks from various fecal sources
Using QMRA, researchers have also investigated the relative risks from different sources of fecal pollution, including human sewage sources, stormwater runoff, bird contamination issues, and agricultural fecal inputs. This research is the basis for recommending the development of site-specific alternative water quality guideline values when appropriate.
Human sources of fecal material (e.g., municipal wastewater, septic systems, other bathers at the beach) pose the greatest risk to human health as they are the sources most likely to contain human pathogens. Most studies have reported that the enteric viruses, specifically norovirus, pose the greatest risk to swimmer's health in recreational waters (Schoen and Ashbolt, 2010; Soller et al., 2010a; Dufour et al., 2012; McBride et al., 2013; Eregno et al., 2016; Vergara et al., 2016). Other fecal sources (e.g., agricultural and wildlife) can contain pathogens of human health concern, but the impacts are more variable. A study of the potential risks from stormwater runoff containing only animal fecal sources estimated the risk to human health from cattle, pig, and chicken manure to range from 30 to 180, from 35 to 65, and from 25 to 6 000 times lower, respectively, than the potential risks from municipal wastewater at similar levels of E. coli and enterococci (Soller et al., 2015). Fresh fecal matter from cattle has been estimated to present a risk of illness similar to municipal wastewater, but chicken, pig and gull feces were lower risk (Soller et al., 2010b). Researchers also estimated at least a 1 log lower health risk from gull feces than from sewage, assuming the same enterococci concentrations (Schoen and Ashbolt, 2010). In recreational sites, enterococci from waterfowl feces can predominate but may represent a significantly lower human health risk compared to sites that have sewage contamination (even at low levels) (Schoen and Ashbolt, 2010). Risk modelling has shown that when less than 10% to 30% of the enterococci present in recreational water is from human sources, the potential risk to human health may be significantly lower than indicated by the bacteriological indicator guideline value (Schoen and Ashbolt, 2010; Soller et al., 2014). Although non-human fecal sources are usually lower risk, there are times when animal fecal material can contain higher levels of human pathogens, for example, E. coli O157:H7 in calves (Soller et al., 2015).
In general, the predominant fecal source at a recreational site may not be the dominant health risk. Some studies have combined QMRA methods with data from human-specific fecal makers (e.g., HF183) to estimate health risks from specific pathogens, as well as to estimate threshold concentrations for the human-specific makers that correspond to the health risk levels associated with E. coli and enterococci guideline values (Brown et al., 2017; Boehm et al., 2018; Boehm and Soller, 2020). Further research on the relative persistence of markers versus infectious pathogens is needed before this work could be used for guideline development.
3.3.3 QMRA in water quality management plans
Although implementation of QMRA requires a high degree of scientific knowledge, it has been used to support site-specific recreational water quality management plans. A pilot project at an Australian beach used sanitary survey information, combined with a QMRA approach, to design a site-specific recreational water quality management program (Ashbolt et al., 2010). In Canada, the Government of Alberta has used published QMRA data on comparative risks to implement fecal source tracking as part of their updated safe beaches protocol (Government of Alberta, 2019). The Guidelines for Canadian Recreational Water Quality – Fecal Indicators technical document uses QMRA study results as support for developing alternative recreational criteria on a site-specific basis (Health Canada, in publication-a). The US EPA, in its most recent recreational water quality criteria, allows for the development of site-specific water quality objectives using QMRA models when non-sewage source(s) drive the health risk (US EPA, 2012).
3.4 Predictive water quality models
Predictive water quality modelling uses mathematical equations to predict whether a water quality target may be exceeded. It can be integrated into recreational water quality management plans to provide an early warning system that health risks may be increased at recreational areas.
Using models to predict fecal indicator bacteria concentrations can overcome some of the limitations of current monitoring approaches, such as the lag times associated with obtaining culture-based fecal indicator bacteria results and less than daily bacteriological sampling frequencies (WHO, 2003; US EPA, 2012, 2016). Predictive models are designed to make same-day predictions, including on weekends, when many recreational areas are not being monitored. Models can also be automated, so that predictions are generated daily and automatically sent to beach managers (Searcy et al., 2018). It should be noted that not all beaches are good candidates for the use of predictive modelling in their recreational water quality management plans (MOE, 2012; US EPA, 2016). Beaches that hardly ever exceed guideline values or beaches where the water quality is known to remain constant over extended periods of time may not benefit from predictive tools. Also, in order for predictive tools to work, a set of variables must be available for use in modelling the water quality with a reasonable degree of accuracy. Recreational areas that are subject to a wide or frequently changing set of conditions and disturbances that are not predictable are not good candidates for modelling.
Predictive models have been developed and validated using data from various water- and weather-related parameters (e.g., rainfall, wave height, wind direction, turbidity, fecal indicator counts). Examples of such models include SwimCast (Olyphant and Pfister, 2005; Lake County Health Department, 2010), NowCast (Francy and Darner, 2007; Francy et al., 2013a; Francy et al., 2013b), and Virtual Beach (Frick et al., 2008; Mednick, 2012; Cyterski et al., 2013; Neet et al., 2015), although numerous other examples have also been published (Nevers et al., 2009; Chan et al., 2013; Francy et al., 2013b; Jones et al., 2013; Gonzalez and Noble, 2014; Brooks et al., 2016; He et al., 2016; US EPA, 2016; Searcy et al., 2018). In many cases, predictive models have been shown to have a degree of accuracy comparable to, or greater than, traditional fecal bacteria beach monitoring for providing correct and timely beach management decisions. Models are not static however, and on-going evaluation and improvements to the models are necessary (Searcy et al., 2018). US EPA (2016) outlines a six-step process for evaluating whether any given beach should consider predictive modelling.
There are a number of challenges associated with using predictive tools. A significant level of technical expertise is required to develop the models, to analyze and interpret the data, and to maintain and re-evaluate the models. Moreover, as mentioned earlier, models may not work in all areas. Modellers use historical data to develop the mathematical equations that describe the relationships between different variables. Therefore, data on the recreational area must be available. Data from well-designed monitoring programs, representative of the range of conditions, will be most useful for developing predictive models. The models need to be validated against monitoring data to ensure they are providing accurate estimates. If these challenges can be addressed, beach operators, service providers or responsible authorities looking for an additional tool with which to potentially improve the timeliness of their water quality decisions may wish to investigate this approach.
3.5 Monitoring for cyanobacteria and their toxins
Appropriate monitoring programs for cyanobacteria blooms and their toxins provide early warning information to inform users of potential health risks. Not all rivers and lakes used for recreational activities need to be monitored for cyanobacteria or their toxins. Instead, responsible authorities should use criteria to help evaluate the risk of bloom formation in order to identify the water bodies that are at greater risk. The types of recreational activities that are taking place in the area and the level of exposure of individuals in the event of a cyanobacteria bloom formation also need to be considered. This information can then be used to prioritize areas that should be monitored, to determine a monitoring approach (e.g., what to monitor, how often), and to develop an action plan for responding to a bloom event. Further guidance on cyanobacteria and their toxins can be found in the Guidelines for Canadian Recreational Water Quality – Cyanobacteria and Their Toxins technical document (Health Canada, 2020).
3.6 Other monitoring
Other water quality parameters (e.g., colour, clarity, oil/grease film) related to the physical or aesthetic characteristics of a recreational water body and its surrounding environment have links to the health and safety of recreational water users and thus may be included in a monitoring program. Guideline values or aesthetic objectives have been specified for these parameters where necessary. Further information can be found in the Guidelines for Canadian Recreational Water Quality – Physical, Aesthetic and Chemical Characteristics technical document (Health Canada, 2021).
4.0 Beach Sand Management
Management of beach sand is important as beach users often spend more time on the beach than in the water, and children routinely play in the sand at the water's edge (DeFlorio-Barker et al., 2018). Beach sands can contain many types of microorganisms, including various pathogens, deposited through numerous routes. This includes direct deposition of fecal materials from bird or animal droppings, human activities on beach sands, runoff from surrounding areas including from storm drains or combined sewer overflows, as well as from the adjacent waters. The concentrations of microorganisms in beach sand can be significantly greater than the adjacent water, but are highly variable over very small distances. Some studies have shown that contact with beach sand can increase the risk of GI illness (Heaney et al., 2009, 2012). Therefore, it is important for users to understand how to minimize this risk (e.g., washing hands before consuming food).
At present, there are no fecal indicator bacteria criteria or guidelines available for assessing sand quality. While studies have investigated different methods for enumerating fecal indicators in sand (Boehm et al., 2009; Staley et al., 2016; Vogel et al., 2017) monitoring of sand samples is not standardized or considered practical and is thus not recommended at this time. However, certain circumstances may warrant testing of sand and sediment samples, such as during investigations of potential waterborne disease outbreaks, after sewage spills, or when conducting an environmental health and safety survey (see Section 2.0).
4.1 Microorganisms in beach sand
Beach sand can harbour microorganisms from fecal matter and other environmental sources. Gulls in particular are thought to be a significant source of fecal indicator bacteria for some beaches (Levesque et al., 1993; Fogarty et al., 2003; Williamson et al., 2004). Their contribution of human pathogenic microorganisms, however, is generally low but does vary depending on contamination sources in the surrounding environment where gulls may also occur (e.g., wastewater management facilities) (Quessy and Messier, 1992; Lu et al., 2011; Alm et al., 2018). Canada geese populations can also present a source of fecal material at areas adjacent to surface waters, and their feces have been found to contain and transport pathogenic viruses, protozoa and bacteria that may pose a human health risk (Moriarty et al., 2011; Gorham and Lee, 2016). Non-fecal sources can include microorganisms naturally found in the sand environment as well as those deposited on the sand through mechanisms such as land runoff and wave action. The numerous sources of microorganisms that may impact beach sands can lead to high concentrations of microbes in the sand, many of which will not be a concern to human health.
Beach sands can provide more favourable conditions for survival of microorganisms than adjacent waters. They can provide protection from sunlight, buffered temperatures, a degree of cover from predation, large surface areas for biofilm development and a replenishing supply of moisture and organic nutrients from wave swash (Whitman and Nevers, 2003; Whitman et al., 2014). When microorganisms are introduced to the beach environment, several outcomes are possible: they may die off within hours, they may persist for days to months without replicating, or some proportion of the bacterial members can become established and start growing in the environment.
The fecal indicator organisms E. coli and enterococci have both exhibited a greater persistence in beach sands relative to natural waters. They have been isolated from numerous locations in the beach environment, including foreshore and nearshore sands (Alm et al., 2003; Whitman and Nevers, 2003; Edge and Hill, 2007; Yamahara et al., 2007, 2012; Staley et al., 2016) and backshore sands (Byappanahalli et al., 2006), as well as from subtropical and temperate stream sediments (Byappanahalli et al., 2003; Jamieson et al., 2003, 2004; Ferguson et al., 2005; Ishii et al., 2006a), temperate forest soils (Byappanahalli et al., 2006), and beach wrack (Whitman et al., 2003; Ishii et al., 2006b). Both E. coli and enterococci may also replicate in beach sands under favourable conditions (Ishii et al., 2006a; Hartz et al., 2008; Yamahara et al., 2009; Halliday and Gast, 2011; Byappanahalli et al., 2012; Whitman et al., 2014). They have also been shown to reach concentrations that are many fold higher than the adjacent swimming waters (Alm et al., 2003; Whitman and Nevers, 2003; Williamson et al., 2004; Bonilla et al., 2007; Edge and Hill, 2007; Halliday and Gast, 2011). Of the beach areas studied, foreshore sands generally have the highest levels of fecal indicator bacteria, potentially resulting from the favourable survival conditions provided by the moist sand or from deposition of fecal droppings by shorebirds (Whitman and Nevers, 2003; Whitman et al., 2006; Whitman et al., 2014; Staley et al., 2016). This is the area where children tend to play in the sand. Although concentrations in beach sand can be significantly greater than the adjacent water, the concentrations are highly variable over very small distances, making beach sand monitoring difficult.
Beach sand can be a reservoir for pathogenic microorganisms that may be a risk to human health. Fecal and non-fecal pathogenic species of bacteria, viruses, parasites and fungi have been isolated from the sand environment (WHO, 2003; Shah et al., 2011). This includes Aeromonas spp., Campylobacter spp., pathogenic E. coli, Pseudomonas aeruginosa, Salmonella spp., Staphylococcus aureus, Vibrio spp., Cryptosporidium spp., Giardia spp., numerous fungal pathogens, and some viral pathogens (Whitman et al., 2014). Of the pathogens studied, Salmonella spp. and Campylobacter spp. are among the most frequently detected (Bolton et al., 1999; Obiri-Danso and Jones, 1999, 2000; Yamahara et al., 2012), with the source most often being related to contamination of the beach sand by bird feces. These pathogens are not expected to grow in beach sand, but can survive for various lengths of time (Eichmiller et al., 2014). S. aureus and P. aeruginosa have also been detected in some studies (Mohammed et al., 2012; Thapaliya et al., 2017). Humans are the primary source of S. aureus as it is not considered a natural inhabitant of environmental waters, whereas P. aeruginosa is widely distributed in the aquatic environment. Both of these non-enteric pathogens may survive and replicate in beach sands (Mohammed et al., 2012). Pathogenic protozoa (Abdelzaher et al., 2010; Shah et al., 2011), enteroviruses (Shah et al., 2011), and other infectious viruses, including the influenza A virus (Poulson et al., 2017), have also been isolated from sands in various marine beaches, but a plausible pathway to human infection is probably limited to the enteric viruses. Multiple pathogenic fungal species have also been identified in sand samples at marine beaches (Sabino et al., 2011).
To date, there has been no consistent relationship between the concentrations of fecal indicator organisms and the occurrence of pathogens in beach sands (Whitman et al., 2014).
4.2 Effects of beach sand on microbiological water quality
There have been numerous studies showing that beach sand can present a significant non-point source of fecal indicator bacteria for swimming waters (Alm et al., 2003; Whitman and Nevers, 2003; Williamson et al., 2004; Yamahara et al., 2007; Edge and Hill, 2007; Heaney et al., 2014; Torres-Bejarano et al., 2018). Disturbances to foreshore and nearshore sands and sediments can affect the microbial quality of shallow waters (WHO, 2003; Skalbeck et al., 2010; Vogel et al., 2017). These disturbances can resuspend fecal indicator microorganisms, inflating microbiological counts. Edge and Hill (2007) applied microbial source tracking techniques and found that sand could be a source of E. coli out to 150 m off a beach in Hamilton Harbour. Mechanisms of transfer of sandborne contamination to the water environment include wave swash, sand erosion, rain-mediated runoff and direct transfer from swimmers (Boehm et al., 2004; Vogel et al., 2016). A study examining the movement of enterococci through sand showed that these organisms were not strongly bound to beach sand and can be easily transferred to the water environment (Yamahara et al., 2007). Resuspension of nearshore sediments can occur through a number of mechanisms, including wave action (including those artificially generated by commercial and recreational boating), storms and swimmer activities. Boehm et al. (2004) proposed that the wave- and tide-driven recirculation of water through the beach aquifer may also present a mechanism for transfer of microorganisms and nutrients from the sand environment to swimming waters. The potential health risks that may be associated with resuspension of microorganisms from beach sand is not currently known.
4.3 Relationship between beach sand and human illness
Several studies have been undertaken to determine whether exposure to beach sand (digging in sand, buried in sand) increases the risk of becoming ill. Whitman et al. (2009) looked at the transferability of E. coli and F+ coliphages from beach sand to hands to assess whether sufficient quantities of microorganisms could be transferred to make it a possible route of exposure to pathogens. They determined that if beach sands contain high levels of human pathogens, the quantities of organisms transferred on the fingertips and hands from playing in the sand could be sufficient to increase the risk of GI illness. This study also reaffirmed that hand washing is effective at removing these microorganisms (i.e., 92% to 98% reduction), supporting the recommendation to always wash your hands before consuming food.
Heaney et al. (2009) studied associations between sand exposure and illness at marine and freshwater beaches under the US EPA's NEEAR study. The authors observed that digging in sand was associated with a modest increase in the risk of GI illness (1.13; CI = 1.02–1.25) and diarrhea (1.20; CI = 1.05–1.36). Individuals who reported being buried in sand showed a slightly higher incidence of GI illness (1.23; CI = 1.05–1.43) and diarrhea (1.24; CI = 1.01–1.52), with children under 10 years old having the strongest association. The increased risks for sand exposure were found for both swimmers and non-swimmers. No associations were demonstrated for sand contact and non-enteric illness (Heaney et al., 2009). In a follow-up study at two recreational marine beaches, Heaney et al. (2012) determined that individuals who reported digging in sand, where enterococci concentrations were in the highest tertile (i.e., the highest third of the ordered distribution; >324 CCE/g of sand), had increased risks of GI illness (2.0: CI = 1.2–3.2) and diarrhea (2.4; CI = 1.4–4.2). Estimates were even higher for GI illness (3.3; CI = 1.3–7.9) and diarrhea (4.9; CI = 1.8–13) among individuals buried in sand. However, the study had very few participants who reported sand exposure but did not swim, so the risk from swimming could not be separated from the risk from beach sand. An earlier study by Marino et al. (1995) reported no evidence of a relationship between the incidence of skin symptoms and sand concentrations of any of the indicator organisms monitored (E. coli, fecal streptococci, Candida albicans, dermatophytic fungi) during a prospective epidemiological study at two beaches in Malaga, Spain. Using a QMRA approach, Shibata and Solo-Gabriele (2012) calculated the concentration of pathogens (Cryptosporidium, enteroviruses, S. aureus) in beach sand that would represent an equivalent level of risk to US EPA water quality recreational limits. They determined that at a non-point source recreational beach in Florida, the concentration of pathogens in the beach sand generally presented a low infection risk.
Further research is needed to more fully characterize the relationships between fecal indicator bacteria and the possible presence of fecal pathogens in beach sand, as well as the potential implications to human health.
5.0 Beach Management Best Practices
Combining management actions, procedures, and tools into beach best management practices can collectively reduce the risks in beach sands and recreational waters, thereby protecting the health of recreational water users. Enteric pathogens are of significant concern in recreational environments, and implementing barriers to reduce the extent of fecal contamination is therefore recommended. An environmental health and safety survey is an important tool for helping recreational water operators identify potential onshore sources of fecal contamination that are relevant to their beach area (see Section 2.0). Removal of litter that may attract animals to the area and the installation of physical barriers (e.g., animal-proof refuse containers, fences and gull nets) designed to discourage wildlife may be useful strategies. Jurisdictional regulations restricting access of pets to public beaches present another potential control mechanism.
Physical manipulation of the sand environment may also help minimize fecal contamination and reduce its transport to swimming waters (Kinzelman et al., 2004; Kinzelman and McLellan, 2009; Hernandez et al., 2014; Whitman et al., 2014; Kelly et al., 2018; Edge et al., 2018). This could include deep mechanical grooming to remove harmful materials, beach berm construction to prevent stormwater runoff onto a beach, and targeted beach grading to increase the steepness of the slope, which reduces the area vulnerable to wave swash and permits more rapid sand drying through improved drainage.
Some beaches may also implement pre-emptive beach postings or swimming advisories restricting recreational water activities for short periods immediately after rainfall events. These limit swimmer exposures to fecal contamination that may have been washed into the swimming or beach area. Not all beaches are negatively impacted by rainfall events, so beach managers should determine whether pre-emptive beach postings will be beneficial for the recreational area. The decision to use pre-emptive beach postings should be based on historical monitoring results and the EHSS. Larger-scale management options for beaches require a comprehensive review of the contamination inputs and watershed characteristics and the identification of specific options to minimize or control the sources of fecal contamination and to reduce the transfer of pollution to the swimming area.
Beach users can also do their part by properly disposing of their litter, refraining from feeding animals on or near the beach, using available facilities for hygiene practices (e.g., washing hands, changing diapers) and complying with any existing beach regulations or codes of conduct. They may also contribute by becoming informed of, and following, steps that can be taken to reduce their personal exposure (see Section 6.0).
Authorities may wish to consult the following types of resources for further information to help them address specific beach-related issues:
- Published texts: Texts are available that provide comprehensive discussion of broader topics like stormwater management, wastewater treatment, and coastal water management. Texts are also available for specific topics such as differentiating plants (like duckweed) from cyanobacteria.
- Searchable databases of journal articles: Keyword searches can direct the user to specific scientific studies, related articles, bibliographical citations and topic review papers.
- Proceedings from international conferences: A review of proceedings can identify accounts of actions evaluated in other communities, providing an indication of results and potential contacts. Specific conferences include the Great Lakes Beach Association Conferences (www.greatlakesbeachassociation.org/) (www.great-lakes.net/glba), and the US EPA National Beach Conferences (www.epa.gov).
- Manuals or publications produced by stakeholder organizations.
Water quality issues can have impacts across multiple fields (e.g., health, environment, agriculture, municipal infrastructure) and require cross-sectoral collaboration. Consultation with responsible authorities, other beach operators or service providers and recreational water quality professionals may help to identify actions that have proven to be successful in other communities.
6.0 Public Awareness and Communication
To participate in safe, enjoyable recreational water activities, the public needs access to information on the quality of the area and its facilities, as well as notification of any existing water quality hazards. Beach operators, service providers and responsible authorities have a responsibility to inform and educate the public and provide adequate warnings about any hazards relevant to their recreational water areas. The public can also do their part by taking actions to protect themselves, such as;
- avoid swallowing water, regardless of the water quality;
- avoid swimming when you have open sores or wounds;
- wash hands before consuming food;
- use clean beach towels to reduce the degree of sand contact; and
- shower after swimming.
The public should also be aware of where the water quality monitoring results are posted and should consult this information before going to the beach.
Efforts to improve the public's awareness and understanding of water quality can have numerous benefits (Bartram and Rees, 2000; Pendleton et al., 2001). Communication tools can be used to:
- reduce the potential risk of swimmer illness or injury;
- improve the quality of the water;
- correct public misconceptions regarding water quality;
- improve public confidence; and
- increase beach attendance.
6.1 Posting of information at recreational water areas
Information on the expected risks associated with the recreational area should be communicated to the user through the use of posted signs. Other means of communicating information to users is provided in Section 6.2. Signs should be posted in locations that are highly visible to the public. The information provided should be easily understood and not open to misinterpretation. Ideally, signs should be standardized to permit comparisons across different locations. Examples of informative beach signs are provided in Appendix C.
Signs and messaging should be used to warn users about the potential risks associated with a recreational area. Even in waters considered to be of good quality for swimming, there is always some risk that swimmers may experience adverse health effects. Warnings should be issued in a timely manner and promptly removed once the issuing authority has determined that the risk no longer exists.
The information provided on warning signs and in messaging should include, at a minimum:
- a statement identifying the health or safety risk;
- recommended actions to be taken;
- the name of the authority responsible for issuing the warning; and
- contact information for the issuing authority.
Warning signs or messages may be posted to announce a swimming advisory, to announce a beach closure, or to provide cautionary information such as permanent signage at beaches with re-occurring cyanobacterial blooms. A swimming advisory or a beach closure should be issued by the medical officer of health or other appropriate authority in accordance with the legislation in each province or territory. This decision should be based on a thorough assessment of the situation with information provided by recreational water monitoring, the EHSS and public health surveillance.
A swimming advisory can be issued if the responsible authority decides that there is an unacceptable level of risk associated with recreational use. Under this situation, users are advised to refrain from whole body contact with the water. Contact with the beach is usually permissible, and access to the facilities is generally not restricted. Examples of scenarios that may trigger jurisdictions to issue a swimming advisory include:
- exceedance of the guideline values for the recommended fecal indicators;
- exceedance of the guideline values for toxic cyanobacteria and their toxins, or in the event of the development of a cyanobacteria bloom;
- evidence of the risk of swimmer's itch for recreational water users; and
- periods of significant rainfall, which could trigger an advisory as a pre-emptive action (at applicable beaches).
A beach closure may be issued (where possible) if the responsible authority determines that a beach or body of water poses a serious risk to the health and safety of recreational water users and that it is necessary to further restrict individuals from coming in contact with the area. Under a closure, the area is considered closed to all recreational activity. Users are advised to avoid contact with the beach and recreational water area, and access to the facilities may be restricted. Examples of situations in which jurisdictions may deem it necessary to issue a beach closure include:
- suspicion that the area is responsible for a waterborne disease outbreak;
- a sewage or chemical spill that is expected to affect the recreational water area; and
- other conditions such that the area is judged to pose a significant risk to public health (e.g., persistently poor water quality; a pandemic).
Appropriate signs may also be posted at waters that have been deemed suitable for secondary contact activities (e.g., rowing, sailing, canoe touring, fishing), but not for primary contact uses (e.g., swimming, wading, windsurfing, waterskiing). In these instances, it may be necessary to expand the location of the signs beyond the beach area to improve their visibility. Suggested locations include relevant points of entry and launch areas. Responsible authorities should also routinely update the signage content, especially for signage in areas with chronic issues (e.g., cyanobacteria blooms) to avoid message fatigue among public users.
6.2 Other tools for public education and communication
In addition to posted signs, other tools can be used to promote public education and communicate information, including:
- printed materials (e.g., posters, information sheets, educational bulletins, pamphlets, brochures);
- media sources such as websites (e.g., Swim Guide – https://www.theswimguide.org/), social media platforms, local newspapers, television and radio announcements;
- participation in beach certification or award programs (e.g., Blue Flag certification);
- educational events such as volunteer monitoring programs and beach cleanup days; and
- classification or grading systems for beaches.
Classification or grading systems for beaches can be used as a tool to promote communication and understanding of water quality information. They are also thought to help encourage a sense of shared accountability and responsibility among the beach authorities and beach users. A number of jurisdictions and multinational organizations have adopted grading systems as part of their recreational water management recommendations (WHO, 2003; NHMRC, 2008; MELCC, 2019). Both the World Health Organization (WHO) and the Australian Guidelines (WHO, 2003; NHMRC, 2008) make use of a grade-based format for fecal indicator density within their framework for assessing fecal pollution in recreational waters. These approaches use a microbiological assessment component along with a sanitary inspection categorization to produce a classification of an area's overall suitability for recreation. Under the Quebec framework for fecal pollution assessment (MDDEP, 2004; MELCC, 2019), water quality grades are assigned to recreational waters based on average yearly fecal indicator monitoring results. This grade is used as a public communication tool and also dictates the sampling frequency requirements for the area.
There are advantages and disadvantages associated with the use of beach grading systems. Responsible authorities should be aware of the limitations of any system when investigating its use as a potential communication tool. For example, grading systems based on fecal indicator monitoring results provide information on only one aspect of recreational water safety. The accuracy of any such grading system would be strongly affected by the limitations known to be associated with fecal indicator monitoring. Ideally, a successful beach grading system would involve criteria from a number of categories, encompassing monitoring results, communication tools and water quality hazard control actions.
7.0 Public Health Advice
Consultation with public health authorities is an essential component of risk management. In the event of an incident (microbiological, chemical or physical) that represents a risk to public health or safety, health officials can play a key role by providing advice to beach managers and determining what actions need to be taken. Local public health authorities should be promptly notified of any situation that threatens the health or safety of recreational water users. There is a particular need for good communication between public health agencies and agencies with responsibilities for managing wastewater infrastructure since changes to sanitary or stormwater systems can have immediate impacts on some beaches. As part of normal operations, local public health officials may be periodically consulted for information and advice on topics pertinent to safe recreational water use.
In assessing the risks associated with recreational water quality hazards, the local health authorities should, wherever possible, establish surveillance for swimmer illness or injuries. This can be established by consulting public health surveillance mechanisms currently in operation or by conducting specific investigations. Information sources include:
- federal, provincial/territorial or regional departments or agencies' surveillance programs or reporting systems;
- clinical reports from hospital emergency departments and local physicians;
- accident or incident reports held by recreational water area operators or service providers;
- formal epidemiological investigations; and
- other potential surveillance mechanisms (e.g., monitoring of over-the-counter medicine sales in pharmacies).
Procedures for the investigation of illness associated with recreational waters should adhere to the recommendations given in the third edition of Procedures to Investigate Waterborne Illness (IAFP, 2016).
8.0 References
Abdelzaher, A.M., Wright, M.E., Ortega, C., Solo-Gabriele, H.M., Miller, G., Elmir, S., Newman, X., Shih, P., Bonilla, J.A., Bonilla, T.D., Palmer, C.J., Scott, T., Lukasik, J., Harwood, V.J., McQuaig, S., Sinigalliano, C., Gidley, M., Plano, L.R.W., Zhu, X., Wang, J.D. and Fleming, L.E. (2010). Presence of pathogens and indicator microbes at a non-point source subtropical recreational marine beach. Appl. Environ. Microbiol., 76(3): 724-732.
Ahmed, W., Goonetilleke, A., Powell, D. and Gardner, T. (2009). Evaluation of multiple sewage-associated Bacteroides PCR markers for sewage pollution tracking. Water Res., 43: 4872-4877.
Ahmed, W., Goonetilleke, A. and Gardner, T. (2010). Human and bovine adenoviruses for the detection of source-specific fecal pollution in coastal waters in Australia. Water Res., 44: 4662-4673.
Ahmed, W., Zhang, Q., Kozak, S., Beale, D., Gyawali, P., Sadowsky, M.J. and Simpson, S. (2019). Comparative decay of sewage-associated marker genes in beach water and sediment in a subtropical region. Water Res., 149: 511-521.
Alm, E.W., Burke, J. and Spain, A. (2003). Fecal indicator bacteria are abundant in wet sand at freshwater beaches. Water Res., 37: 3978-3982.
Alm, E.W., Daniels-Witt, Q.R., Learman, D.R., Ryu, H., Jordan, D.W., Gehring, T.M. and Santo Domingo, J. (2018). Potential for gulls to transport bacteria from human waste sites to beaches. Sci. Total Environ., 615: 123-130.
Ashbolt, N.J., Schoen, M.E., Soller, J.A. and Roser, D.J. (2010). Predicting pathogen risks to aid beach management: The real value of quantitative microbial risk assessment (QMRA). Water Res., 44: 4692-4703.
Bartram, J. and Rees, G. (eds.). (2000). Monitoring bathing waters - A practical guide to the design and implementation of assessments and monitoring programmes. New York (NY): E & FN Spon.
Bernhard, A.E. and Field, K.G. (2000a). Identification of nonpoint sources of fecal pollution in coastal waters by using host-specific 16S ribosomal DNA genetic markers from fecal anaerobes. Appl. Environ. Microbiol., 66: 1587-1594.
Bernhard, A.E. and Field, K.G. (2000b). A PCR assay to discriminate human and ruminant feces on the basis of host differences in Bacteroides-Prevotella genes encoding 16S rRNA. Appl. Environ. Microbiol., 66: 4571-4574.
Bertke, E.E. (2007). Composite analysis for Escherichia coli at coastal beaches. J. Great Lakes Res., 33(2): 335-341.
Boehm, A.B., Grant, S.B., Kim, J.H., Mowbray, S.L., McGee, C.D., Clark, C.D., Foley, D.M. and Wellman, D.E. (2002). Decadal and shorter period variability of surf zone water quality at Huntington Beach, California. Environ. Sci. Technol., 36: 3885-3892.
Boehm, A.B., Fuhrman, J.A., Mrše, R.D. and Grant, S.B. (2003). Tiered approach for identification of a human fecal pollution source at a recreational beach: case study at Avalon Bay, Catalina Island, California. Environ. Sci. Technol., 37: 673-680.
Boehm, A.B., Shellenbarger, G.G. and Paytan, A. (2004). Groundwater discharge: potential association with fecal indicator bacteria in the surf zone. Environ. Sci. Technol., 38: 3558-3566.
Boehm, A.B., Griffith, J., McGee, C., Edge, T.A., Solo-Gabriele, H.M., Whitman, R., Cao, Y., Getrich, M., Jay, J.A., Ferguson, D., Goodwin, K.D., Lee, C.M., Madison, M. and Weisberg, S.B. (2009). Faecal indicator bacteria enumeration in beach sand: a comparison study of extraction methods in medium to coarse sands. J. Appl. Microbiol., 107: 1740-1750.
Boehm, A. B., Soller, J. A. and Shanks, O. C. (2015) Human-associated fecal quantitative polymerase chain reaction measurements and simulated risk of gastrointestinal illness in recreational waters contaminated with raw sewage. Environ. Sci. Technol. Lett., 2 (10): 270-275.
Boehm, A.B., Graham, K.E. and Jennings, W. (2018). Can we swim yet? Systematic review, meta-analysis, and risk assessment of aging sewage in surface waters. Environ. Sci. Technol., 52(17): 9634-9645.
Boehm, A.B. and Soller, J.A. (2020). Refined ambient water quality thresholds for human-associated fecal indicator HF183 for recreational waters with and without co-occurring gull fecal contamination. Microb. Risk Anal., 16: 100139.
Bolton, F.J., Surman, S.B., Martin, K., Wareing, D.R. and Humphrey, T.J. (1999). Presence of Campylobacter and Salmonella in sand from bathing beaches. Epidemiol. Infect., 122(1): 7-13.
Bonilla, T.D., Nowosielski, K., Cuvelier, M., Hartz, A., Green, M., Esiobu, N., McCorquodale, D.S., Fleisher, J.M. and Rogerson, A. (2007). Prevalence and distribution of fecal indicator organisms in South Florida beach sand and preliminary assessment of health effects associated with beach sand exposure. Mar. Pollut. Bull., 54: 1472-1482.
Bower, P.A., Scopel, C.O., Jensen, E.T., Depas, M.M. and McLellan, S.L. (2005). Detection of genetic markers of fecal indicator bacteria in Lake Michigan and determination of their relationship to Escherichia coli densities using standard microbiological methods. Appl. Environ. Microbiol., 71: 8305-8313.
Brooks, W., Corsi, S., Fienen, M. and Carvin, R. (2016). Predicting recreational water quality advisories: A comparison of statistical methods. Environ. Model. Softw., 76: 81-94.
Brown, K.I., Graham, K.E., Soller, J.A. and Boehm, A.B. (2017). Estimating the probability of illness due to swimming in recreational water with a mixture of human- and gull-associated microbial source tracking markers. Environ. Sci. Process. Impacts, 19(12): 1528-1541.
Byappanahalli, M., Fowler, M., Shively, D. and Whitman, R. (2003). Ubiquity and persistence of Escherichia coli in a Midwestern coastal stream. Appl. Environ. Microbiol., 69(8): 4549-4555.
Byappanahalli, M.N., Whitman, R.L. Shively, D.A., Sadowsky, M.J. and Ishii, S. (2006). Population structure, persistence, and seasonality of autochthonous Escherichia coli in temperate, coastal forest soil from a Great Lakes watershed. Environ. Microbiol., 8(3): 504-513.
Byappanahalli, M.N., Nevers, M.B., Korajkic, A., Staley, Z.R. and Harwood, V.J. (2012). Enterococci in the environment. Microbiol. Mol. Biol. Rev., 76(4): 685-706.
Cao, Y., Sivaganesan, M., Kelty, C.A., Wang, D., Boehm, A.B., Griffith, J.F., Weisberg, S.B. and Shanks, O.C. (2018). A human fecal contamination score for ranking recreational sites using
the HF183/BacR287 quantitative real-time PCR method. Water Res., 128: 148-156.
Carson, A.C., Shear, B.L., Ellersieck, M.R. and Asfaw, A. (2001). Identification of fecal Escherichia coli from humans and animals by ribotyping. Appl. Environ. Microbiol., 67: 1503-1507.
CCME (2004). From source to tap: Guidance on the multi-barrier approach to safe drinking water. Produced jointly by the Federal-Provincial-Territorial Committee on Drinking Water and the Canadian Council of Ministers of the Environment Water Quality Task Group. Available at: www.hc-sc.gc.ca/ewh-semt/water-eau/drink-potab/multi-barrier/index-eng.php.
Chan, S.N., Thoe, W. and Lee, J.H.W. (2013). Real-time forecasting of Hong Kong beach water quality by 3D deterministic model. Water Res., 47: 1631-1647.
Codd, G.A., Morrison, L.F. and Metcalf, J.S. (2005). Cyanobacterial toxins: risk management for health protection. Toxicol. Appl. Pharmacol., 203(3): 264-272.
Converse, R.R., Kinzelman, J.L., Sams, E.A., Hudgens, E., Dufour, A.P., Ryu, H., Santo-Domingo, J.W., Kelty, C.A., Shanks, O.C., Siefring, S.D., Haugland, R.A. and Wade, T.J. (2012). Dramatic improvements in beach water quality following gull removal. Environ. Sci. Technol., 46(18): 10206-10213.
Cyterski, M., Brooks, W., Galvin, M., Wolfe, K., Carvin, R., Roddick, T., Fienen, M. and Corsi, S. (2013). Virtual Beach 3.0.4: User’s Guide. National Exposure Research Laboratory, U.S. Environmental Protection Agency, Athens, GA and U.S. Geological Survey, Middleton, WI.
DeFlorio-Barker, S., Arnold, B.F., Sams, E.A., Dufour, A.P., Colford, J.M., Jr., Weisberg, S.B., Schiff, K.C. and Wade, T.J. (2018). Child environmental exposures to water and sand at the beach: Findings from studies of over 68,000 subjects at 12 beaches. J. Expo. Sci. Environ. Epidemiol., 28(2): 93-100.
Devane, M.L., Moriarty, E.M., Robsona, B., Lin, S., Wood, D., Webster-Brown, J. and Gilpin, B.J. (2019). Relationships between chemical and microbial faecal source tracking markers in urban river water and sediments during and post-discharge of human sewage. Sci. Total Environ., 651: 1588-1604.
Dombek, P.E., Johnson, L.K., Zimmerley, S.T. and Sadowsky, M.J. (2000). Use of repetitive DNA sequences and the PCR to differentiate Escherichia coli isolates from human and animal sources. Appl. Environ. Microbiol., 66: 2572-2577.
Dorevitch, S., Dworkin, M.S., DeFlorio, S.A., Janda, W.M., Wuellner, J. and Hershow, R.C. (2012). Enteric pathogens in stool samples of Chicago-area water recreators with new-onset gastrointestinal symptoms. Water Res., 46: 4961-4972.
Dufour, A., Wade, T.J. and Kay, D. (2012). Epidemiological studies on swimmer health effects associated with potential exposure to zoonotic pathogens in bathing beach water – a review. In: Dufour, A., Bartram, J., Bos, R. and Gannon, V. (eds.). Animal waste, water quality and human health. London (UK): IWA Publishing, pp. 415-428.
Edge, T.A. and Hill, S. (2007). Multiple lines of evidence to identify the sources of fecal pollution at a freshwater beach in Hamilton Harbour, Lake Ontario. Water Res., 41(16): 3585-3594.
Edge, T.A., Hill, S., Seto, P. and Marsalek, J. (2010). Library-dependent and library-independent microbial source tracking to identify spatial variation in fecal contamination sources along a Lake Ontario beach (Ontario, Canada). Water Sci. Technol., 62(3): 719-727.
Edge, T.A., Hill, S., Crowe, A., Marsalek, J., Seto, P., Snodgrass, B., Toninger, R. and Patel, M. (2018). Remediation of a beneficial use impairment at Bluffer’s Park Beach in the Toronto Area of Concern. Aquat. Ecosyst. Health Manag., 21: 285-292.
Edge, T.A., Boyd, R.J., Shum, P. and Thomas, J.L. (2021). Microbial source tracking to identify fecal sources contaminating the Toronto Harbour and Don River watershed in wet and dry weather. J. Great Lakes Res. (in press).
Eichmiller, J.J., Borchert, A.J., Sadowsky, M.J. and Hicks, R.E. (2014). Decay of genetic markers for fecal bacterial indicators and pathogens in sand from Lake Superior. Water Res., 59: 99-111.
Eregno, F.E., Tryland, I., Tjomsland, T., Myrmel, M., Robertson, L. and Heistad, A. (2016). Quantitative microbial risk assessment combined with hydrodynamic modelling to estimate the public health risk associated with bathing after rainfall events. Sci. Total Environ., 548-549: 270-279.
Farkas, K., Adriaenssens, E.M., Walker, D.I., McDonald, J.E., Malham, S.K. and Jones, D.L. (2019). Critical evaluation of crAssphage as a molecular marker for human-derived wastewater contamination in the aquatic environment. Food Environ. Virol., 11: 113-119.
Ferguson, D.M., Moore, D.F., Getrich, M.A. and Zhowandai, M.H. (2005). Enumeration and speciation of enterococci found in marine and intertidal sediments and coastal water in southern California. J. Appl. Microbiol., 99(3): 598-608.
Fogarty, L.R., Haack, S.K., Wolcott, M.J. and Whitman, R.L. (2003). Abundance and characteristics of the recreational water quality indicator bacteria Escherichia coli and enterococci in gull faeces. J. Appl. Microbiol., 94(5): 865-878.
Francy, D.S. and Darner, R.A. (2007). Nowcasting beach advisories at Ohio Lake Erie beaches: U.S. Geological Survey Open-File Report 2007–1427, 13 p.
Francy, D.S., Brady, A.M.G., Carvin, R.B., Corsi, S.R., Fuller, L.M., Harrison, J.H., Hayhurst, B.A., Lant, J., Nevers, M.B., Terrio, P.J. and Zimmerman, T.M. (2013a). Developing and implementing predictive models for estimating recreational water quality at Great Lakes beaches: U.S. Geological Survey Scientific Investigations Report 2013–5166, 68 p.
Francy, D.S., Stelzer, E.A., Duris, J.W., Brady, A.M.G., Harrison, J.H., Johnson, H.E. and Ware, M.W. (2013b). Predictive models for Escherichia coli concentrations at inland lake beaches and relationship of model variables to pathogen detection. Appl. Environ. Microbiol., 79(5): 1676.
Frick, W.E., Ge, Z. and Zepp, R.G. (2008). Nowcasting and forecasting concentrations of biological contaminants at beaches: a feasibility and case study. Environ. Sci. Technol., 42(13): 4818-4824.
Glassmeyer, S.T., Furlong, E.T., Kolpin, D.W., Cahill, J.D., Zaugg, S.D., Werner, S.L., Meyer, M.T. and Kryak, D.D. (2005). Transport of chemical and microbial compounds from known wastewater discharges: potential for use as indicators of human fecal contamination. Environ. Sci. Technol., 39(14): 5157-5169.
Gonzalez, R.A. and Noble, R.T. (2014). Comparisons of statistical models to predict fecal indicator bacteria concentrations enumerated by qPCR- and culture-based methods. Water Res., 48: 296-305.
Gorham, T.J. and Lee, J. (2016). Pathogen loading from Canada geese faeces in freshwater: Potential risks to human health through recreational water exposure. Zoonoses Public Health, 63: 177-190.
Government of Alberta (2019). Alberta safe beach protocol. July 2019.
Green, H.C., Dick, L.K., Gilpin, B., Samadpour, M. and Field, K.G. (2012). Genetic markers for rapid PCR-based identification of gull, Canada goose, duck, and chicken fecal contamination in water. Appl. Environ. Microbiol., 78(2): 503-510.
Green, H.C., Haugland, R.A., Varma, M., Millen, H.T., Borchardt, M.A., Field, K.G., Walters, W.A., Knight, R., Sivaganesan, M., Kelty, C.A. and Shanks, O.C. (2014). Improved HF183 quantitative real-time PCR assay for characterization of human fecal pollution in ambient surface water samples. Appl. Environ. Microbiol., 80(10): 3086-3094.
Griffith, J.F., Weisburg, S.B. and McGee, C.D. (2003). Evaluation of microbial source tracking methods using mixed fecal sources in aqueous test samples. J. Water Health, 1(4): 141-151.
Griffith, J.F., Layton, B.A., Boehm, A.B., Holden, P.A., Jay, J.A., Hagedorn, C., McGee, C.D. and Weisberg, S.B. (2013). The California microbial source identification manual: a tiered approach to identifying fecal pollution sources at beaches. Southern California Coastal Water Research Project. Technical Report 804. December, 2013.
Haack, S.K., Duris, J.W., Fogarty, L.R., Kolpin, D.W., Focazio, M.J., Furlong, E.T. and Meyer, M.T. (2009). Comparing wastewater chemicals, indicator bacteria concentrations, and bacterial pathogen genes as fecal pollution indicators. J. Environ. Qual., 38: 248-258.
Halliday, E. and Gast, R.J. (2011). Bacteria in beach sands: an emerging challenge in protecting coastal water quality and bather health. Environ. Sci. Technol., 45(2): 370-379.
Hartz, A., Cuvelier, M., Nowosielski, K., Bonilla, T.D., Green, M., Esiobu, N., McCorquodale, D.S. and Rogerson, A. (2008). Survival potential of Escherichia coli and Enterococci in subtropical beach sand: implications for water quality managers. J. Environ. Qual., 37(3): 898-905.
Harwood, V.J., Staley, C. Badgley, B.D., Borges, K. and Korajkic, A. (2014). Microbial source tracking markers for detection of fecal contamination in environmental waters: relationships between pathogens and human health outcomes. FEMS Microbiol. Rev., 38: 1-40.
He, C., Post, Y., Dony, J., Edge, T., Patel, M. and Rochfort, Q. (2016). A physical descriptive model for predicting bacteria level variation at a dynamic beach. J. Water Health, 14(4): 617.
Health Canada (2020). Guidelines for Canadian recreational water quality – cyanobacteria and their toxins. Guideline Technical Document for Public Consultation.
Health Canada (2021). Guidelines for Canadian recreational water quality – physical, aesthetic and chemical characteristics. Guideline Technical Document for Public Consultation.
Health Canada. (in publication-a). Guidelines for Canadian recreational water quality: Guideline Technical Document – Indicators of Fecal Contamination. Water and Air Quality Bureau, Healthy Environments and Consumer Safety Branch, Health Canada, Ottawa, Ontario.
Health Canada. (in publication-b). Guidelines for Canadian recreational water quality: Guideline Technical Document – Microbiological Methods. Water and Air Quality Bureau, Healthy Environments and Consumer Safety Branch, Health Canada, Ottawa, Ontario.
Heaney, C.D., Sams, E., Wing, S., Marshall, S., Brenner, K., Dufour, A.P. and Wade, T.J. (2009). Contact with beach sand among beachgoers and risk of illness. Am. J. Epidemiol., 170(2): 164-172. Epub 2009 Jun 18.
Heany, C.E., Sams, E., Dufour, A.P., Brenner, K.P., Haugland, R.A., Chern, E., Wing, S., Marshall, S., Love, D.C., Serre, M., Noble, R. and Wade, T.J. (2012). Fecal indicators in sand, sand contact, and risk of enteric illness among beachgoers. Epidemiology, 23(1): 95-106.
Heaney, C.D., Exuma, N.G., Dufour, A.P., Brenner, K.P., Haugland, R.A., Chern, E. Schwab, K.J., Love, D.C., Serre, M.L., Noble, R. and Wade, T.J. (2014). Water quality, weather and environmental factors associated with fecal indicator organism density in beach sand at two recreational marine beaches. Sci. Total Environ., 497-498: 440-447.
Hernandez, R.J., Hernandez, Y., Jimenez, N.H., Piggot, A.M., Klaus, J.S., Feng, Z., Reniers, A. and Solo-Gabriele, H.M. (2014). Effects of full-scale beach renovation on fecal indicator levels in shoreline sand and water. Water Res. 48: 579-591.
Hughes, B., Beale, D.J., Dennis, P.J., Cook, S. and Ahmed, W. (2017). Cross-comparison of human wastewater-associated molecular markers in relation to fecal indicator bacteria and enteric viruses in recreational beach waters. Appl. Environ. Microbiol., 83(8): e00028-17.
IAFP (2016). Procedures to investigate waterborne illness. 3rd edition. International Association for Food Protection, Committee on the Control of Foodborne Illness, Des Moines, Iowa.
Ishii, S., Ksoll, W.B., Hicks, R.E. and Sadowsky, M.J. (2006a). Presence and growth of naturalized Escherichia coli in temperate soils from Lake Superior watersheds. Appl. Environ. Microbiol., 72(1): 612-621.
Ishii, S., Yan, T., Shively, D.A., Byappanahalli, M.N., Whitman, R.L. and Sadowsky, M.J. (2006b). Cladophora (Chlorophyta) spp. harbor human bacterial pathogens in nearshore water of Lake Michigan. Appl. Environ. Microbiol., 72(7): 4545-4553.
Jamieson, R.C., Gordon, R.J. and Tattrie, S.C. (2003). Sources and persistence of fecal coliform bacteria in a rural watershed. Water Qual. Res. J. Canada, 38(1): 33-47.
Jamieson, R.C., Joy, D.H., Lee, H., Kostachuk, R. and Gordon, R.J. (2004). Persistence of enteric bacteria in alluvial streams. J. Environ. Eng. Sci., 3: 203-212.
Johnston, C., Ufnar, J.A., Griffith, J.F., Gooch, J.A. and Stewart, J.R. (2010). A real-time qPCR assay for the detection of the nifH gene of Methanobrevibacter smithii, a potential indicator of sewage pollution. J. Appl. Microbiol., 109: 1946-1956.
Jones, R.M., Liu, L. and Dorevitch, S. (2013). Hydrometeorological variables predict fecal indicator bacteria densities in freshwater: data-driven methods for variable selection. Environ. Monit. Assess., 185: 2355-2366.
Kelly, E.A., Feng, Z., Gidley, M.L., Sinigalliano, C.D., Kumar, N., Donahue, A.G., Reniers, A.J.H.M. and Solo-Gabriele, H.M. (2018). Effect of beach management policies on recreational water quality. J. Environ. Manage., 212: 266-277.
Khatib, L.A., Tsai, Y.L. and Olson, B.H. (2002). A biomarker for the identification of cattle fecal pollution in water using the LTIIa toxin gene from enterotoxigenic Escherichia coli. Appl. Microbiol. Biotechnol., 59: 97-104.
Khatib, L.A., Tsai, Y.L. and Olson, B.H. (2003). A biomarker for the identification of swine fecal pollution in water, using the STII toxin gene from enterotoxigenic Escherichia coli. Appl. Microbiol. Biotechnol., 63: 231-238.
Kildare, B.J., Leutenegger, C.M., McSwaina, B.S., Bambic, D.G, Rajala, V.B. and Wuertz, S. (2007). 16S rRNA-based assays for quantitative detection of universal, human-, cow-, and dog-specific fecal Bacteroidales: A Bayesian approach. Water Res., 41: 3701-3715.
Kinzelman, J.L., Pond, K.R., Longmaid, K.D. and Bagley, R.C. (2004). The effect of two mechanical beach grooming strategies on Escherichia coli density in beach sand at a southwestern Lake Michigan beach. Aquat. Ecosyst. Health Manag., 7(3): 425-432.
Kinzelman, J.L., Dufour, A.P., Wymer, L.J., Rees, G., Pond, K.R. and Bagley, R.C. (2006). Comparison of multiple point and composite sampling for monitoring bathing water quality. Lake Reserv. Manag., 22(2): 95-102.
Kinzelman, J.L. and McLellan, S.L. (2009). Success of science-based best management practices in reducing swimming bans—a case study from Racine, Wisconsin, USA. Aquat. Ecosyst. Health Manag., 12(2): 187-196.
Korajkic, A., McMinn, B., Herrmann, M.P., Sivaganesan, M., Kelty, C.A., Clinton, P., Nash, M.S. and Shanks, O.C. (2020). Viral and bacterial fecal indicators in untreated wastewater across the contiguous United States exhibit geospatial trends. Appl. Environ. Microbiol., 86: e02967-19.
Lake County Health Department (2010). SwimCast data. Lake County Health Department, Waukegan, Illinois. Available at: www.lakecountyil.gov.
Layton, A., McKay, L., Williams, D., Garrett, V., Gentry, R. and Sayler, G. (2006). Development of Bacteroides 16S rRNA gene TaqMan-based real-time PCR assays for estimation of total, human, and bovine fecal pollution in water. Appl. Environ. Microbiol., 72(6): 4214-4224.
Leecaster, M.K. and Weisberg, S.B. (2001). Effect of sampling frequency on shoreline microbiology assessments. Mar. Pollut. Bull., 42: 1150-1154.
Levesque, B., Brousseau, P., Simard, P., Dewailly, E., Meisels, M., Ramsay, D. and Joly, J. (1993). Impact of the Ring-billed Gull (Larus delawarensis) on the microbiological quality of recreational waters. Appl. Environ. Microbiol., 59(4): 1228-1230.
Lu, J., Santo Domingo, J.W., Lamendella, R., Edge, T. and Hill, S. (2008). Phylogenetic diversity and molecular detection of bacteria in gull feces. Appl. Environ. Microbiol., 74(13): 3969-3976.
Lu, J., Ryu, H., Santo Domingo, J.W., Griffith, J.F. and Ashbolt, N. (2011). Molecular detection of Campylobacter spp. in California gull (Larus californicus) excreta. Appl. Environ. Microbiol., 77(14): 5034-5039.
Marino, F., Moringo, M., Martinez-Manzanares, E. and Borrego, J. (1995). Microbiological-epidemiological study of selected marine beaches in Malaga (Spain). Water Sci. Technol., 31: 5-9.
Mayer, R.E., Reischer, G.H., Ixenmaier, S.K., Derx, J., Blaschke, A.P., Ebdon, J.E., Linke, R., Egle, L., Ahmed, W., Blanch, A.R., Byamukama, D., Savill, M., Mushi, D., Cristóbal, H.A., Edge, T.A., Schade, M.A., Aslan, A., Brooks, Y.M., Sommer, R., Masago, Y., Sato, M.I., Huw, Å., Taylor, D., Rose, J.B., Wuertz, S., Shanks, O.C., Piringer, H., Mach, R.L., Savio, D., Zessner, M. and Farnleitner, A.H. (2018). Global distribution of human-associated fecal genetic markers in reference samples from six continents. Environ. Sci. Technol., 52(9): 5076-5084.
McBride, G.B., Stott, R., Miller, W., Bambic, D. and Wuertz, S. (2013). Discharge-based QMRA for estimation of public health risks from exposure to stormwater-borne pathogens in recreational waters in the United States. Water Res., 47: 5282-5297.
McQuaig, S.M., Scott, T.M., Lukasik, J.O., Paul, J.H. and Harwood, V.J. (2009). Quantification of human polyomaviruses JC virus and BK virus by TaqMan quantitative PCR and comparison to other water quality indicators in water and fecal samples. Appl. Environ. Microbiol., 75(11): 3379-3388.
MDDEP (2004). Critères de qualité de l’eau de surface au Québec. Ministère du Développement durable, de l’Environnement et des Parcs. Government of Quebec. Available at: www.mddep.gouv.qc.ca/eau/criteres_eau/index.htm.
MELCC (2019). La qualité de l’eau et les usages récréatifs. Ministère de l’Environnement et de la Lutte contre les changements climatiques. Government of Quebec
Mednick, A.C. (2012). Building operational “Nowcast” models for predicting water quality at five Michigan beaches. Wisconsin Department of Natural Resources, Madison, WI. PUB-SS-1098 2012.
MOE (2012). Technical bulletin: Is your beach a candidate for predictive modeling? Ministry of the Environment. Government of Ontario. Toronto, ON [unpublished].
Mohammed, R.L, Echeverry, A., Stinson, C.M., Green, M., Bonilla, T.D., Hartz, A., McCorquodale, D.S., Rogerson, A. and Esiobu, N. (2012). Survival trends of Staphylococcus aureus, Pseudomonas aeruginosa, and Clostridium perfringens in a sandy South Florida beach. Mar. Pollut. Bull., 64: 1201-1209.
Moriarty, E.M., Karki, N., Mackenzie, M., Sinton, L.W., Wood, D.R. and Gilpin, B.J. (2011). Fecal indicators and pathogens in selected New Zealand waterfowl. N. Z. J. Mar. Freshwater Res., 45(4): 679-688.
Napier, M.D., Haugland, R., Poole, C., Dufour, A.P., Stewart, J.R., Weber, D.J., Varma, M., Lavender, J.S. and Wade, T.J. (2017). Exposure to human-associated fecal indicators and self-reported illness among swimmers at recreational beaches: a cohort study. Environ. Health, 16: 103.
Napier, M.D., Poole, C., Stewart, J.R., Weber, D.J., Glassmeyer, S.T., Kolpin, D.W., Furlong, E.T., Dufour, A.P. and Wade, T.J. (2018). Exposure to human-associated chemical markers of fecal contamination and self-reported illness among swimmers at recreational beaches. Environ. Sci. Technol., 52(13): 7513-7523.
Neet, M.J., Kelsey, R.H., Porter, D.E., Ramage, D.W. and Jones, A.B. (2015) Model results and software comparisons in Myrtle Beach, SC using Virtual Beach and R regression toolboxes. Journal of South Carolina Water Resources, 2(1): Article 8.
Nevers, M.B., Shively, D.A., Kleinheinz, G.T., McDermott, C.M., Schuster, W., Chomeau, V. and Whitman, R.L. (2009). Geographic relatedness and predictability of Escherichia coli along a peninsular beach complex of Lake Michigan. J. Environ. Qual., 38: 2357-2364.
Nguyen, K.H., Senay, C., Young, S., Nayak, B., Lobos, A., Conrad, J. and Harwood, V.J. (2018). Determination of wild animal sources of fecal indicator bacteria by microbial source tracking (MST) influences regulatory decisions. Water Res., 144: 424-434.
NHMRC (2008). Guidelines for managing risks in recreational water. National Health and Medical Research Council of Australia, Government of Australia, Canberra.
Noble, R.T., Griffith, J.F., Blackwood, A.D., Fuhrman, J.A., Gregory, J.B., Hernandez, X., Liang, X., Bera, A.A. and Schiff, K. (2006). Multitiered approach using quantitative PCR to track sources of fecal pollution affecting Santa Monica Bay, California. Appl. Environ. Microbiol., 72: 1604-1612.
Obiri-Danso, K. and Jones, K. (1999). Distribution and seasonality of microbial indicators and thermophilic campylobacters in two freshwater bathing sites on the River Lune in northwest England. J. Appl. Microbiol., 87(6): 822-832.
Obiri-Danso, K. and Jones, K. (2000). Intertidal sediments as reservoirs for hippurate negative campylobacters, salmonellae and fecal indicators in three EU recognised bathing waters in north west England. Water Res., 34(2): 519-527.
Okabe, S., Okayama, N., Savichtcheva, O. and Ito, T. (2007). Quantification of host-specific Bacteroides–Prevotella 16S rRNA genetic markers for assessment of fecal pollution in freshwater. Appl. Microbiol. Biotechnol., 74: 890-891.
Olyphant, G.A. and Pfister, M. (2005). SwimCast: Its physical and statistical basis. In
Proceedings of the Joint Conference—Lake Michigan: State of the Lake and the Great
Lakes Beach Association, Green Bay, WI, November 2–3, 2005.
Palmer, J.A., Law, J.Y. and Soupir, M.L. (2020). Spatial and temporal distribution of E. coli contamination on three island lake and recreational beach systems in the upper Midwestern United States. Sci. Total Environ., 722:137846.
Pendleton, L., Martin, N. and Webster, D.G. (2001). Public perceptions of environmental quality: a survey study of beach use and perceptions in Los Angeles County. Mar. Pollut. Bull., 42: 1155-1160.
Poulson, R.L., Luttrell, P.M., Slusher, M.J., Wilcox, B.R., Niles, L.J., Dey, A.D., Berghaus, R.D., Krauss, S., Webster, R.G. and Stallknecht, D.E. (2017). Influenza A virus: sampling of the unique shorebird habitat at Delaware Bay, USA. R. Soc. Open Sci., 4: 171420.
Quessy, S. and Messier, S. (1992). Prevalence of Salmonella spp., Campylobacter spp. and Listeria spp. in ring-billed gulls (Larus delawarensis). J. Wildl. Dis., 28(4): 526-531.
Reicherts, J.D. and Emerson, C.W. (2010). Monitoring bathing beach water quality using composite sampling. Environ. Monit. Assess., 168: 33-43.
Rijal, G., Tolson, J.K., Petropoulou, C., Granato, T.C., Glymph, A., Gerba, C., Deflaun, M.F., O’Connor, C., Kollias, L. and Lanyon, R. (2011). Microbial risk assessment for recreational use of the Chicago Area Waterway System. J. Water Health, 9(1): 169-186.
Rusiñol, M., Fernandez-Cassi, X., Hundesa, A., Vieira, C., Kern, A., Eriksson, I., Ziros, P., Kay, D., Miagostovich, M., Vargha, M., Allard, A., Vantarakis, A., Wyn-Jones, P., Bofill-Mas, S. and Girones, R. (2014). Application of human and animal viral microbial source tracking tools in fresh and marine waters from five different geographical areas. Water Res., 59: 119-129.
Sabino, R., Veríssimo, C., Cunha, M.A., Wergikoski, B., Ferreira, F.C., Rodrigues, R., Parada, H., Falcão, L., Rosado, L., Pinheiro, C., Paixão, E. and Brandão, J. (2011). Pathogenic fungi: An unacknowledged risk at coastal resorts? New insights on microbiological sand quality in Portugal. Mar. Pollut. Bull., 62: 1506-1511.
Schoen, M.E. and Ashbolt, N.J. (2010). Assessing pathogen risk to swimmers at non-sewage impacted recreational beaches. Environ. Sci. Technol., 44: 2286-2291.
Scott, T.M., Jenkins, T.M., Lukasik, J. and Rose, J.B. (2005). Potential use of a host associated molecular marker in Enterococcus faecium as an index of human fecal pollution. Environ. Sci. Technol., 39: 283-287.
Searcy, R.T., Taggart, M., Gold, M. and Boehm, A.B. (2018). Implementation of an automated beach water quality nowcast system at ten California oceanic beaches. J. Environ. Manage., 223: 633-643.
Shah, A.H., Abdelzaher, A.M., Phillips, M., Hernandez, R., Solo-Gabriele, H.M., Kish, J., Scorzetti, G., Fell, G.W., Diaz, M.R., Scott, T.M., Lukasik, J., Harwood, V.J., McQuaig, S., Sinigalliano, C.D., Gidley, M.L., Wanless, D., Ager, A., Lui, J., Stewart, J.R., Plano, L.R.W. and Fleming, L.E. (2011). Indicator microbes correlate with pathogenic bacteria, yeasts and helminthes in sand at a subtropical recreational beach site. J. Appl. Microbiol., 110: 1571-1583.
Shanks, O.C., Atikovic, E., Blackwood, A.D., Lu, J., Noble, R.T., Santo Domingo, J., Seifring, S., Sivaganesan, M. and Haugland, R.A. (2008). Quantitative PCR for detection and enumeration of genetic markers for bovine fecal pollution. Appl. Environ. Microbiol., 74(3): 745-752.
Shanks, O.C., Kelty, C.A., Sivaganesan, M., Varma, M. and Haugland, R.A. (2009). Quantitative PCR for genetic markers of human fecal pollution. Appl. Environ. Microbiol., 75(17): 5507-5513.
Shibata, T. and Solo-Gabriele, H.M. (2012). Quantitative microbial risk assessment of human illness from exposure to marine beach sand. Environ. Sci. Technol., 46: 2799-2805.
Skalbeck, J.D., Kinzelman, J.L. and Mayer, G.C. (2010). Fecal indicator organism density in
beach sands: impact of sediment grain size, uniformity, and hydrologic factors
on surface water loading. J. Gt. Lakes Res. 36 (4): 707-714.
Soller, J.A., Bartrand, T., Ashbolt, N.J., Ravenscroft, J. and Wade T.J. (2010a). Estimating the primary etiologic agents in recreational freshwaters impacted by human sources of fecal contamination. Water Res., 44: 4736-4747.
Soller, J.A., Schoen, M.E., Bartrand, T., Ravenscroft, J.E. and Ashbolt, N.J. (2010b). Estimated human health risks from exposure to recreational waters impacted by human and non-human sources of fecal contamination. Water Res., 44: 4674-4691.
Soller, J.A., Schoen, M.E., Varghese, A., Ichida, A.M., Boehm, A.B., Eftim, S., Ashbolt, N.J. and Ravenscroft, J.E. (2014). Human health risk implications of multiple sources of fecal indicator bacteria in a recreational waterbody. Water Res., 66: 254-264.
Soller, J., Bartrand, T., Ravenscroft, J., Molina, M., Whelan, G., Schoen, M. and Ashbolt, N. (2015). Estimated human health risks from recreational exposures to stormwater runoff containing animal fecal material. Environmental Modelling Software, 72: 21-32.
Soller, J.A., Eftim, S., Wade, T.J., Ichida, A.M., Clancy, J.L., Johnson, T.B., Schwab, K., Ramirez-Toro, G., Nappier, S. and Ravenscroft, J.E. (2016). Use of quantitative microbial risk assessment to improve interpretation of a recreational water epidemiological study. Microbial Risk Anal., 1: 2-11.
Soller, J.A., Schoen, M., Steele, J.A., Griffith, J.F. and Schiff, K.C. (2017). Incidence of gastrointestinal illness following wet weather recreational exposures: Harmonization of quantitative microbial risk assessment with an epidemiologic investigation of surfers. Water Res., 121: 280-289.
Staley, Z.R., Robinson, C. and Edge, T.A. (2016). Comparison of the occurrence and survival of fecal indicator bacteria in recreational sand between urban beach, playground and sandbox
settings in Toronto, Ontario. Sci. Total Environ., 541: 520-527.
Staley, C., Kaiser, T., Lobos, A., Ahmed, W., Harwood, V.J., Brown, C.M. and Sadowsky, M.J. (2018a). Application of SourceTracker for accurate identification of fecal pollution in recreational freshwater: a double-blinded study. Environ. Sci. Technol., 52: 4207-4217.
Staley, Z.R., Boyd, R.J., Shum, P. and Edge, T.A. (2018b). Microbial source tracking using quantitative and digital PCR to identify sources of fecal contamination in stormwater, river water, and beach water in a Great Lakes Area of Concern. Appl. Environ. Microbiol., 84(20): e01634-18.
Stoeckel, D.M., Mathes, M.V., Hyer, K.E., Hagedorn, C., Kator, H., Lukasik, J., O’Brien, T.L., Fenger, T.W., Samadpour, M., Strickler, K.M. and Wiggins, B.A. (2004). Comparison of seven protocols to identify fecal contamination sources using Escherichia coli. Environ. Sci. Technol., 38: 6109-6117.
Stoeckel, D.M. (2005). Selection and application of microbial source tracking tools for water-quality investigations. United States Geological Survey, United States Department of the Interior. 43 pp. Techniques and Methods 2-A3
Symonds, E.M., Sinigalliano, C., Gidley, M., Ahmed, W., McQuaig-Ulrich, S.M. and Breitbart, M. (2016). Fecal pollution along the southeastern coast of Florida and insight into the use of pepper mild mottle virus as an indicator. J. Appl. Microbiol., 121: 1469-1481.
Thapaliya, D., Dalman, M., Kadariya, J., Little, K., Mansell, V., Taha, M.Y., Grenier, D. and Smith, T.C. (2017). Characterization of Staphylococcus aureus in good feces from state parks in Northeast Ohio. EcoHealth, 14: 303-309.
Torres-Bejarano, F., González-Márquez, L.C., Díaz-Solano, B., Torregroza-Espinosa, A.C. and Cantero-Rodelo, R. (2018). Effects of beach tourists on bathing water and sand quality at Puerto Velero, Colombia. Environ. Dev. Sustain., 20(1): 255-269.
Tran, N.H., Yew-Hoong Gin, K. and Ngo, H.H. (2015). Fecal pollution source tracking toolbox for identification, evaluation and characterization of fecal contamination in receiving urban surface waters and groundwater. Sci. Total Environ., 538: 38-57.
Unno, T., Staley, C., Brown, C.M., Han, D., Sadowsky, M.J. and Hur, H. (2018). Fecal pollution: new trends and challenges in microbial source tracking using next-generation sequencing. Environ. Microbiol., 20(9): 3132-3140.
US EPA (2005a). The EMPACT Beaches Project: results from a study on microbiological monitoring in recreational waters. National Exposure Research Laboratory, Office of Research and Development, United States Environmental Protection Agency, Cincinnati, Ohio.
US EPA (2005b). Microbial source tracking guide. Office of Research and Development, United States Environmental Protection Agency, Cincinnati, Ohio. 133 pp. (EPA/600-R-05-064).
US EPA (2008). Great Lakes beach sanitary survey user manual. United States Environmental Protection Agency, Office of Water, Document Number: EPA-823-B-06-001,
US EPA (2010). Sampling and consideration of variability (temporal and spatial) for monitoring of recreational waters. United States Environmental Protection Agency, Office of Water, Document Number: EPA-820-F-12-058.
US EPA (2012). Recreational water quality criteria. United States Environmental Protection Agency, Office of Water, Document Number: EPA-820-B-13-001.
US EPA (2013). Marine beach sanitary survey user manual. United States Environmental Protection Agency, Office of Water, Document Number: EPA-820-B-13-001.
US EPA (2016). Six key steps for developing and using predictive tools at your beach. United States Environmental Protection Agency, Office of Water, Document Number: EPA-820-R-16-001.
US EPA (2019). Method 1696: Characterization of Human Fecal Pollution in Water by HF183/BacR287 TaqMan® Quantitative Polymerase Chain Reaction (qPCR) Assay. US EPA Office of Water. EPA 821-R-19-002. 56 p.
Vergara, G.G.R.V., Rose, J.B. and Gin, K.Y.H. (2016). Risk assessment of noroviruses and human adenoviruses in recreational surface waters. Water Res., 103: 276-282.
Vogel, L.J., O’Carroll, D.M., Edge, T.A. and Robinson, C.E. (2016). Release of Escherichia coli from foreshore sand and pore water during intensified wave conditions at a recreational beach. Environ. Sci. Technol., 50(11): 5676-5684.
Vogel, L.J., Edge, T.A., O'Carroll, D.M., Solo-Gabriele, H.M., Kushnir, C.S.E. and Robinson, C.E. (2017). Evaluation of methods to sample fecal indicator bacteria in foreshore sand and pore water at freshwater beaches. Water Res., 121: 204-212.
Weidhaas, J.L., Macbeth, T.W., Olsen, R.L., Sadowsky, M.J., Norat, D. and Harwood, V.J. (2010). Identification of a Brevibacterium marker gene specific to poultry litter and development of a quantitative PCR assay. J. Appl. Microbiol., 109: 334-347.
Whitman, R.L. and Nevers, M.B. (2003). Foreshore sand as a source of Escherichia coli in nearshore water of a Lake Michigan beach. Appl. Environ. Microbiol., 69(9): 5555-5562.
Whitman, R.L., Shively, D.A., Pawlik, H., Nevers, M.B. and Byappanahalli, M.N. (2003). Occurrence of Escherichia coli and enterococci in Cladophora (Chlorophyta) in nearshore water and beach sand of Lake Michigan. Appl. Environ. Microbiol., 69: 4714-4719.
Whitman, R.L. and Nevers, M.B. (2004). Escherichia coli sampling reliability at a frequently closed Chicago beach: monitoring and management implications. Environ. Sci. Technol., 38: 4241-4246.
Whitman, R.L., Nevers, M.B. and Byappanahalli, M.N. (2006). Examination of the watershed-wide distribution of Escherichia coli along Southern Lake Michigan: an integrated approach. Appl. Environ. Microbiol. 72(11): 7301-7310.
Whitman, R.L., Przybyla-Kelly, K., Shively, D.A., Nevers, M.B. and Byappanahalli, M.N. (2009). Hand-mouth transfer and potential for exposure to E.coli and F+ coliphage in beach sand, Chicago, Illinois. J. Water Health, 7(4): 623-629
Whitman, R.L., Harwood, V.J., Edge, T.A., Nevers, M.B., Byappanahalli, M., Vijayavel, K., Brandão, J., Sadowsky, M.J., Wheeler Alm, E., Crowe, A, Ferguson, D., Ge, Z., Halliday, E., Kinzelman, J., Kleinheinz, G., Przybyla-Kelly, K., Staley, C., Staley, Z. and Solo-Gabriele, H.M. (2014). Microbes in beach sands: integrating environment, ecology and public health. Rev. Environ. Sci. Biotechnol., 13: 329-368.
WHO (1999). Health-based monitoring of recreational waters: the feasibility of a new approach (the “Annapolis Protocol”). Outcome of an expert consultation, Annapolis, MD, co-sponsored by the U.S. Environmental Protection Agency. World Health Organization, Geneva, Switzerland (WHO/SDE/WDH/99.1).
WHO (2003). Guidelines for safe recreational water environments. Vol. 1. Coastal and fresh waters. World Health Organization, Geneva, Switzerland.
WHO (2004). Guidelines for drinking-water quality. 3rd edition. World Health Organization, Geneva, Switzerland.
WHO (2009). Addendum to the guidelines for safe recreational water environments, Vol. 1. Coastal and fresh waters. World Health Organization, Geneva, Switzerland.
Wiggins, B.A. (1996). Discriminant analysis of antibiotic resistance patterns in fecal streptococci, a method to differentiate human and animal sources of fecal pollution in natural waters. Appl. Environ. Microbiol., 62: 3997-4002.
Williamson, D.A., Ralley, W.E., Bourne, A., Armstrong, N., Fortin, R. and Hughes, C.E. (2004). Principal factors affecting Escherichia coli at Lake Winnipeg beaches, Manitoba, Canada—interim report. Manitoba Water Stewardship, Winnipeg, Manitoba. 18 pp. (Manitoba Water Stewardship Report No. 2004-01).
Wong, K., Fong, T., Bibby, K. and Molina, M. (2012). Application of enteric viruses for fecal pollution source tracking in environmental waters. Environ. Int., 45: 151-164.
Yamahara, K.M., Layton, B.A., Santoro, A.E. and Boehm, A.B. (2007). Beach sands along the California coast are diffuse sources of fecal bacteria to coastal waters. Environ. Sci. Technol., 41: 4515-4521.
Yamahara, K.M., Walters, S.P. and Boehm, A.B. (2009). Growth of enterococci in unaltered, unseeded beach sands subjected to tidal wetting. Appl. Environ. Microbiol., 75(6): 1517-1524.
Yamahara, K.M., Sassoubre, L.M., Goodwin, K.D. and Boehm, A.B. (2012). Occurrence and persistence of bacterial pathogens and indicator organisms in beach sand along the California coast. Appl. Environ. Microbiol., 78(6): 1733-1745.
Appendix A: List of abbreviations
CCE/g - Calibrator cell equivalents per gram
EHSS - Environmental health and safety survey
GI - Gastrointestinal illness
GIS - Geographic information system
MST - Microbial source tracking
PCR - Polymerase chain reaction
QMRA - Quantitative microbial risk assessment
qPCR - Quantitative polymerase chain reaction
US EPA - United States Environmental Protection Agency
WHO - World Health Organization
Appendix B: Recreational swimming area environmental health and safety survey (EHSS) checklist
Identification
- Beach Name:
- Address:
- Responsible Authority:
- Tel.:
- Fax:
- E-mail:
- Person(s) Conducting Survey:
- Date :
- Time:
Background Information
- Water Body Type:
- Dimmensions of Beach
Length(m):
Width(m): - Dimmensions of Swimming Area
Length(m):
Width(m): - Number of Sampling Sites:
[Attach Map or Aerial Photo of Suitable Scale (including location of sample sites)] - Water Temperature
High/Low(°C):
Average(°C): - Prevailing Winds
Direction:
Avg. speed(km/h): - Prevailing Currents
Direction:
Avg. speed(km/h): - Rainfall
Total(mm):
24-h High(mm): - Wave Height
Average(m):
Range(m):
Use of surrounding area (check all that apply)
- Urban
- Rural
- Agriculture(specify)
- Residential
- Forest
- Commercial(specify)
- Field
- Hills/Uplands
- Industrial(specify)
- Marsh/Swamp
- River/Stream/Ditch
- Harbour
- Other(specify)
Microbiological Hazards
Potential Sources of Fecal Contamination
- Municipal Sewage Discharges
- Combined Sewer Overflows (CSOs)
- Stormwater Drains/Discharges
- Septic Waste Systems
- Wastes from Animal Feeding
- Other
- Other Discharges Containing Fecal Wastes (List)
- Other Sewage Collection/Disposal/ Treatment Systems (List)
Stormwater Runoff from
- Agricultural Areas
- Beach and Surrounding Facilities(e.g. parking)
- Areas Receiving Sewage Sludge
- Other
Other Environmental Sources
(#’s: None Low Med High [circle one for each])
- Discharging Rivers/Streams/Creeks
- Birds(e.g., gulls, ducks, geese, other)
- Other wild animals
- Pets
- Swimmers
- Other
Items for Consideration During the Risk Assessment
- Proximity of potential contamination sources to the swimming area.
- Potential for contamination sources to have an impact on the swimming area (including an indication of their risk priority: Low, Medium, High).
- Evaluation of water quality according to historical microbiological data (e.g., frequency of exceedances of the guideline values for the recommended indicators of fecal contamination [e.g., continuous/periodic/sporadic]).
- Discharges: Assessment of such factors as volume, flow rate, treatment type, applicable indicator standards, periodicity (continuous, sporadic) and predictability.
- Effects of rainfall: Levels triggering contamination events and typical event duration.
- Assessment of swimming area circulation: Effect of onshore winds, tides, currents, flow patterns in transporting fecal contamination to and entrapping it within the swimming area.
- Animals and birds: Assessment of their types, numbers and droppings.
- Impact of swimmers on water quality—numbers, ages.
- Assessment of potential barriers: Barrier types and points at which they may be applied to reduce impact of the contamination source and/or swimmer exposure.
Chemical Hazards
Possible sources of chemical contamination
- Commercial/Industrial Discharges
- Marinas
- Motorized Watercraft
- Other
Stormwater Runoff from the following
- Areas subject to Pesticide Application
- Areas subject to Fertilizer Application
- Urban Areas
- Other
Items for Consideration During the Risk Assessment
- Proximity of potential contamination sources to the bathing area.
- Potential for contamination sources to have an impact on the swimming area (including an indication of their risk priority: Low, Medium, High).
- Discharges: Assessment of such factors as volume, flow rate, treatment type, periodicity (continuous, sporadic) and predictability.
- Effects of rainfall: Levels triggering contamination events and typical event duration.
- Assessment of swimming area circulation: Effect of onshore winds, tides, currents, flow patterns in potentially transporting chemical contamination to and entrapping it within the swimming area.
- Motorized watercraft: Assessment of their types and numbers.
- Assessment of potential barriers: Barrier types and points at which they may be applied to reduce impact of the contamination source and/or swimmer exposure.
Other Biological Hazards
Other Biological Hazards Known to Affect the Recreational Water Area
(Presence may be continuous, seasonal or sporadic.)
- Cyanobacterial Blooms
- Schistosomes(Swimmer’s Itch)
- Large Numbers of Aquatic Plants
- Other(specify)
Items for Consideration During the Risk Assessment
- Seasonal nature of the hazard: continuous, annual, sporadic.
- Presence of contributing factors (as applicable): water conditions, local geography, temperatures, nutrient levels, presence of appropriate host species.
- Assessment of potential barriers to control hazard and/or reduce human exposure in areas/during times of increased risk.
Physical Hazards and Aesthetic Considerations
Subsurface Hazards
- Steep Slopes or Drop-offs
- Depths Greater than 4.5 m
- Large Rocks
- Slippery or Uneven Bottom
- Other
Water Conditions
- Strong Currents or Riptides
- Undertows
Other
(None Low Med High [circle one for each])
- Litter on Beach
- Floating Debris
- Broken Glass or Other Sharp Objects
- Medical Wastes
- Seaweed/Algae on Beach
Vehicles Permitted on Beach or Near Bathing Area
- Automobiles : Y/N
- Boats/Watercraft : Y/N
- Specify :
Items for Consideration During the Risk Assessment
- Assessment of the physical characteristics of the beach and their potential impacts on safe enjoyable use of the area; includes evaluation of physical layout (geography, topography), composition of shoreline and bottom material, influence of existing structures.
- Assessment of potential risks posed by specific hazards/factors in causing injury or illness or otherwise interfering with the enjoyable use of the area.
- Shoreline and water free from obstructions and of sufficient clarity to permit viewing of persons who may be in distress.
- Assessment of the nature and origin of litter and floating debris.
- Applicable physical and aesthetic parameters (pH, temperature, turbidity, colour, clarity, litter) in agreement with recommendations given in the Guidelines for Canadian Recreational Water Quality.
- Assessment of potential barriers to control hazard and/or reduce human exposure in areas/during times of increased risk.
Facilities and Safety Provisions
Facilities
- Number of Toilets:
- Number of Showers:
- Number of Drinking Water Fountains:
- Number of Litter Bins:
- Number of Access for Persons with Disabilities:
- Number of Other:
Safety Provisions
- Number of Lifeguard Stations:
- Number of Emergency Contact Information:
- Number of Emergency Telephone:
- Number of First Aid Stations:
Signs/Communication Materials
- Beach Posting/Suitability for Swimming
- Emergency Contact Information
- Other Hazards (list)
Formal Procedures or Reporting Mechanisms in Place to Deal with the following
- Municipal or Industrial Spills/Discharges/Treatment Bypasses
- Waterborne Disease Outbreaks
- Swimmer Injuries
Items for Consideration During the Risk Assessment
- Assessment of the adequacy of facilities and safety provisions.
- Evaluation of signs and other materials for public communication: Message clear and concise, signs placed in locations highly visible to the public.
Appendix C: Informative beach signs

C.1: Beach posting: example of an informative swimming advisory sign
This figure shows an example of an informative swimming advisory sign, which communicates that the water is not suitable for swimming. It clearly shows that a risk exists, instructs users regarding the suitability of the water for swimming, has a graphic that is easy to understand, provides additional information for the public, and displays contact information for the monitoring program and the authority responsible for the advisory.

C.2: Beach posting: example of an informative swimming suitable for swimming sign
This figure shows an example of an informative swimming advisory sign, which communicates that the beach is open. It instructs users regarding the suitability of the water for swimming, has a graphic that is easy to understand, provides additional information for the public, and displays contact information for the monitoring program and the authority responsible for the advisory.
Footnotes
- Footnote 1
-
For the purposes of these Guidelines, 'depth' refers to the vertical distance in the water extending from the bottom of the water to the surface.
Page details
- Date modified: