Agent of Deterioration: Light, Ultraviolet and Infrared
Stefan Michalski
Table of Contents
The Dilemma: Seeing Versus Saving
We need light in order to see collections, but light damages some objects. In terms of risk management trade-offs, we must make a decision that minimizes the loss of value due to poor visual access and the loss of value due to permanent damage. In terms of ethics and visual access, we must balance the rights of our own generation with the rights of all future generations. In terms of practical reality, we must generalize across a multitude of such decisions because objects differ in both their sensitivity to light and their visibility. In addition, display spaces in many museums depend on highly variable and poorly controlled lighting. This section examines the components of these decisions and offers some summary guidelines. However, the painful dilemma never disappears — seeing collections well today, and seeing them "well" in the future.
Quantifying Light, UV, and IR
Light Does Not "Contain" Ultraviolet and Infrared
In the museum business, one often hears the expression "the light contains ultraviolet and infrared." This is incorrect and will lead to unnecessary confusion in practical discussions of museum lighting. Light, by definition, is the band of radiation to which our eye is sensitive. Ultraviolet radiation (UV) and infrared radiation (IR) are not visible. They are the bands of radiation on either side of the visible band (ultra means beyond, infra means below). Informally, the term radiation is dropped. We usually speak of ultraviolet and infrared, or simply of UV and IR. Ultraviolet and infrared are not necessary for seeing (except in rare cases of UV fluorescent colours); therefore, they are not part of the dilemma between seeing and damaging, they are simply damaging.
It is correct, however, to state that some light sources emit ultraviolet and infrared, or that museum lighting may cause UV and IR deterioration
The Radiation Spectrum
Figure 1 plots the adjacent bands of UV, light, and IR on the conventional scale of wavelength (in nanometers – nm). The reciprocal scale for photon energy is also shown (in electron Volts – eV) to show how photon energy climbs rapidly in the direction of the UV band.

Radiation bands emitted by various light sources are shown by light grey bands. Bands of radiation blocked by some filters are shown as dark grey bands. Convention assigns the boundary between UV and light at 400 nm, but slight perception begins at 380 nm. This boundary of 380 nm is often used by the window industry in rating the UV characteristics of glazing.
The different types of damage typical of UV, light, and IR result from their different photon energies. The photochemistry that underlies much of the disintegration of materials and production of yellow by-products typical of UV exposure requires energies greater than about 3 eV, whereas the photochemistry typical of colourant fading, as well as the operation of our retina, occurs in a range between about 2 eV and 3 eV. We are fated, in fact, to see in the same band as that which causes sensitive colourants to fade, given the related photochemical phenomena. Infrared photons are not energetic enough to initiate any of the forms of photochemistry driven by UV or light, so their effect is simply a heating of the surfaces that absorb them.
Measuring Light and its Exposure
The technical term for the amount of light falling on a surface is "illuminance," but informal phrases such as "light intensity" or "lux level" are used in the museum literature. The unit is lux (both singular and plural). Old light meters may still use the imperial unit "foot candles." Their readings can be converted to lux by multiplying them by 10 (10.76 precisely). Many companies make light meters, also called lux meters. Some of these meters are especially designed for museums so that they include UV and even RH and temperature measurement.
Figure 2 plots various situations and their lux levels across the vast range of the human eye, from moonlight to sunlight.
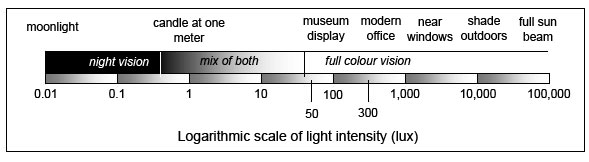
Our eye changes from night vision (scotopic) to colour vision (photopic), with a mixed range (mesopic) between. Rate of light damage is proportional to intensity; therefore, it increases 10 million times from moonlight to sunlight, and 1,000 times from good museum levels to sunlight.
The total exposure or dose of light on a surface is the product of light intensity (lux) and time (hours). In museums, the practical unit is millions of lux hours, abbreviated Mlx h, and pronounced "mega lux hours."
Measuring UV and its Exposure
Rather than measure the intensity of UV directly, the convention in museums has been to measure it relative to the intensity of the light, in units of microwatts (of UV) per lumen (of light), abbreviated µW/lm. This ratio is much more useful than the direct measure of UV when characterizing light sources in a museum and characterizing the benefits of any UV filters on these sources. Various companies make UV meters for museums
Although some authors have suggested doing so, it has not been conventional to measure UV exposure in museums. One can express it if needed as a combination of the light exposure in Mlx h and the UV (ratio) in µW/lm, as will be done later in Table 5, Sensitivity to UV.
Measuring IR
There are no museum conventions or common instruments for measuring IR because it is not nearly as important as UV or light to collection damage. To make a simple instrument for measuring the heating potential of IR from a light source, paint the bulb of an ordinary outdoor glass thermometer with a matte black paint. Place the bulb in the light beam near the object and wait until the temperature stops rising (several minutes). To see if the temperature rise is a problem, refer to the section on Incorrect temperature. As a common-sense alternative estimate, place your hand in the light beam (at the point it might strike artifacts) and use a piece of cardboard to alternately illuminate and shade your palm. If you feel a noticeable warming due to the light, then those artifacts identified as sensitive to "temperature too high" in the section on Incorrect temperature will be at risk.
How Much Light Do We Need to See?
The Benchmark is 50 lux
When guidelines for museum lighting were first explored 60 years ago, colour science had established that 50 lux was enough to ensure that the human eye was operating well within the range of full colour vision (consult Figure 2); therefore, conservation adopted it as the benchmark level for museums. Since then, however, the public has voiced complaints about low light levels in museums. Although our responsibility for the future viewer will always force us to use low light levels for some objects, it is useful to understand the validity of the statement "I cannot see the objects."
A more precise description of our ability to see at 50 lux emerged in the s, centred not simply on whether we could discriminate differences between patches of colour, but whether we could see the tiny details of an object. It emerged that a young person (age 25) viewing a moderately light-coloured object, with a moderate degree of detail, in a moderately complex pattern, in a reasonable period of time, will see all the details almost as well at 50 lux as they will in full sunshine. Unfortunately, they will not see those details as well as they can in sunshine if the object is dark, if the details are very fine, or if the pattern one is looking for within the details is subtle, and the viewing time is limited. Even more unfortunately, someone older (age 65) will need several times as much light to see as well as the youth, even with all necessary optical corrections such as glasses. Recent research has shown that even our ability to discriminate large patches of colour falters as we age.
Adjustments for Everyone to See Better
It is obvious to us all that we see tiny details much better in brighter light, especially if the object is dark, or the details very "soft" (i.e. low contrast), or when one is searching for subtle patterns in these details such as in an etching on handmade paper versus a good facsimile on machine-made paper. Our ability to see objects as real, genuine, and authentic, resides in our ability to see such details. One cannot imagine an institution more devoted to people "seeing the real thing" than a museum; hence, the complaints when they cannot. The question becomes: how much visibility of the real thing should a museum provide, given the steep cost to the lifetime of the objects? And how much more light does this increased visibility require?
Details | Adjustments |
---|---|
Benchmark value, reasonable visibility for young viewer: | 50 lux |
For dark surfaces: | Up to 3 times the lux |
For low contrast details: | Up to 3 times the lux |
For very fine details or complex time-limited task: | Up to 3 times the lux |
For older viewers: | Up to 3 times the lux |
A combination of the above factors: multiply the factors; therefore, up to 3 × 3 × 3 × 3 × 50 lux, for a total of up to ~4,000 lux for an old person looking for subtle patterns in fine detail in a dark object. |
If we use the 50 lux benchmark, Table 1 summarizes some simple (and conservative) rules for adjusting visibility for different objects. For a technical summary of the research underlying these adjustments for visibility and the original sources, consult Michalski .
Table 1 does not imply that a museum must make these adjustments, it simply describes the adjustments necessary to maintain good object visibility across various situations. Whether or not one adopts any of these adjustments for visibility depends on the balance with the preservation issues raised in the later sections on deterioration by light and UV. This balance forms the subject of the final section on "Control Strategies."
Adjustments for Older Viewers to See Equally Well
Our visual system is not so much a still camera as a video camera connected to a complex and dynamic processor. As we age, not only do the lenses in our eyes yellow and fluoresce, but more stray light is created from internal scattering, cones and rods decrease in number, and the neural processing deteriorates. This is above and beyond the issues of normal aging that can be corrected with glasses and age-related pathologies that cannot. The factor of times 3 given in Table 1, to give us equal visual access at age 65 as we have at age 25, is smaller than actually necessary, but it does provide most of the benefit.
Lighting Design Mistakes that Reduce Visibility
How can lighting mistakes reduce visibility, and why does it especially matter in a museum?
The human visual system has a range of many orders of magnitude — the steps in the lux scale of Figure 2 — but at any one moment, given a wide range of colour brightness in one scene, we can only adapt to a fraction of one such step. The three mechanisms involved in adjusting our sensitivity — neural adaptation, iris size adjustment, and photoreceptor chemistry — take between 200 milliseconds and an hour to adjust. In a museum, lighting designs that exceed our eye's ability to adjust over time and space can be considered a mistake. Given the price paid in fading for giving visual access, it makes sense to avoid lighting mistakes that reduce this access.
Direct glare: Block
As with oncoming headlights that dominate our eyes and diminish the visibility of the adjacent road, any bright lamp or window shining in our eyes will diminish the visibility of an object. Direct glare greatly exceeds the sensitivity range of our eye and forces it to adapt to the higher intensity.
Block any such glare: on lamps, use extension tubes ("snoots"), baffles, and louvers; on windows, use shutters, curtains, or blinds. (New blind materials are available that maintain the view, but block almost all the intensity.) Complex exhibition routes with interior partitions and numerous display cases will require many hours of chasing down glare from lamps, re-aiming them, or blocking them. One of the advantages of a simple perimeter wall layout, whether a long 19th-century, barrel-vaulted gallery or a small 20th-century room (consult Vignette 2) is the reduction of such problems.
Reflected or veiling glare: Test it
Display cases and glazed picture frames form one of the most cost-effective preservation strategies in a museum; however, the reflections they cause can become one of the most vexing characteristics of museum displays. Few people can predict reflections from drawings, and few museums will change a display after it is built "just because of reflections." Test before fabricating final designs. Purchase an artist's stretcher, or other wooden frame, and stretch clear plastic wrap over it. Place the frame wherever you plan the display case or the picture under glass; have someone hold utility lamps where you plan the lighting; stand where you expect the visitor to stand; and then check the plastic sheet for any lamp reflections. Some reflection from overhead lighting is unavoidable. The goal is to move it below eye level for even the shortest visitors. The view from a child's height is often disastrous, hence some of their boredom.
Genuine anti-reflection glass is available, but at great cost (the coating is the same as used on camera lenses, computer monitors, and some eyeglasses). It has been used most often in framing important paintings in historic house museums, where avoiding window reflections may become impossible. Low-cost "anti-glare" glass relies on a slightly frosted surface, and only works well if placed directly against the painting; therefore, it is not recommended for museums.
Background contrast: Avoid it
Most old objects look brighter and less damaged when placed on a dark matte surface, than when placed on a bright glossy surface. Try it. The museum tradition of white surfaces everywhere, as somehow "neutral" for display rooms and cases must be re-examined. When judging the effect of "nice bright" walls, one must ask whether the collection itself looks bright, or just the space — at the expense of the objects. Backlit panels in displays, other than providing silhouettes, must be recognized as completely dysfunctional in terms of artifact visibility.
Visual adaptation: Support it
The eye adapts remarkably well to lower levels, but it does take several minutes (as we all know from entering a cinema theatre). Final adaptation can take up to an hour. Many museums that have been conscientious in their gallery lighting suffer from exhibit entrances that appear "closed" because they are so dark compared to the entrance foyer. Consider reducing foyer illumination. Whenever possible, design a transition into exhibit spaces so that visitors can adapt in stages. Perhaps illuminate the introductory didactic panels slightly brighter than the main part of the exhibit space, as an invitation and a transition (though not so bright that it becomes its own adaptation or glare mistake).
Sources of Light, UV, and IR
A "Palette" of Light Sources for Museums
One currently has a daunting range of options for museum lighting. Table 2 summarizes the advantages, disadvantages, costs, and other parameters of currently available light sources.
Characteristic | Incandescent | Fluorescent | |
---|---|---|---|
Traditional | Quartz Halogen | Traditional tubes | |
Voltage | 220 V, 120 V | 220 V, 120 V, 12 V, 6 V | 220 V, 120 V |
Common Types and Nomenclature | A19, R30, R40, PAR38 A: common round bulb R: reflector ER: elliptical reflector PAR: parabolic reflector Number refers to diameter in multiples of 1/8 in. (3 mm). Many manufacturer specialities, e.g. Flurospray. As of , many R4 and PAR types no longer available due to energy legislation. |
MR16, PAR20, PAR30, PAR36 MR: multiple reflector PAR: parabolic reflector Number refers to diameter in multiples of 1/8 in. (3 mm). MR16 types also referred to by three letters, e.g. B.A.B., E.X.N., etc. Q series: no reflector, number refers to wattage. |
T5, T8, T10, T12 T: tube diameter in multiples of 1/8 in. (3 mm). F18, F20, F40, F96 F: fluorescent, number refers to wattage. Colour temperature often given by letter: CW: cool white WW: warm white CWX: cool white deluxe WWX: warm white deluxe "Daylight", and many trademark names for colour temperature |
Lifetime, Hours | A, R, PAR: 2,000, ER: 5,000+ |
2,000 typical, but very short lifetimes in some museum uses such as fibre optics have been reported. Confirm first. | 10,000 typical |
Cost (per lamp) | A: $2 R, PAR, ER: $5-$10 |
$5-$25 | $5-$20 (varies as CRI) |
Relamp Cost (per year of 3,000 hr) | A: $3; R, PAR, ER: $7-$30 |
$8-$40 | $1.5-$6 |
Colour Temp (below 3000 K= warm light) (above 4000 K= cool light) |
2700-2800 K typical, i.e. warm. Flurospray blue filter will increase to approximately 2900 K. |
3,000 K typical, i.e. warm, but cooler than ordinary incandescent. | "warm white": 3000 K "cool white": 4200 K "daylight": 5000-6500 K Others as specified |
Colour Rendering Index (CRI) excellent: 90 – 100 good: 80– 89 fair: 70–79 bad: below 70 |
100, excellent. Dichroic reflectors (low heat) may reduce CRI. Note: incandescent lamps and daylight both score 100 by definition of the CRI because both have a smooth "black body" spectrum, i.e. no missing parts between 400 nm and 760 nm. The low colour temperature of traditional incandescent lamps, however, sometimes provokes criticism in museums, especially for paintings created outdoors using blue colours. The increase from 2800 K to 3000 K with quartz halogen lamps eliminates most such criticism. |
CW, WW: 50–60, bad deluxe: 70–90, fair to good special types: 90–95, excellent |
|
UV Output µW/lm | 75, low | Behind glass: 100–200, medium. Those labelled UV-STOP: 40, low. |
Most 75-150, low to medium A few higher |
UV filter Possibilities | Not essential, but highly UV-sensitive objects would benefit from a UV filter. | Bare quartz bulb emits shortwave UV, blocked by plain glass envelope or safety filter. Museum quality glass UV filters $10-$50. Low-cost plastic filters must be at a distance from the hot bulb. |
Plastic sleeve UV filters available. Ensure end caps are certified against fire risk (some have ignited). Alternatively, place UV filters on fixture diffuser |
Fibre Optic or Lightpipe Application | Inappropriate | MR16 commonly used in fibre optic illuminators. Fibres will filter UV and infrared. Illuminator: $200–$500 From 1-10 separate fibre outputs per fixture typical, sometimes more. |
Lightpipe shelves can be used in cases. |
Main Museum Advantages | "A" types very low cost Fixtures low cost |
Excellent variety of beam widths and wattages available. Best light spectrum overall. Low-voltage lamps can be wired without concern for shock hazard. Very low change in lamp output over lifetime of lamp. |
Low-frequency relamping. Little heat from tube. Low energy consumption. |
Main Museum Disadvantages | Too bright at less than 1.5 m. Highest heat output of any lamps (not suitable inside cases). No narrow spots. |
Bulbs are very hot, bare quartz bulbs can explode. High heat output. Lamp cost per hour can be high. Some low voltage fixtures very expensive. Bare wire designs create fire risk. |
Too bright at short distances. Not easily directed in a beam. Most fixtures look ugly, lighting can be "flat." |
Characteristic | Fluorescent | HID (high intensity discharge) | White LED (light emitting diode) | Daylight |
---|---|---|---|---|
Compact | ||||
Voltage | 220 V, 120 V | 220 V, 120 V and higher | 6 V, 12 V, 120 V, 220 V | Not Available |
Common Types and Nomenclature | CFT: compact fluorescent tube. Manufacturers may use other letters, e.g. TL, XL, PL, SL. Sizes: 5W, 7W, 9W, 11W, 13W, etc. where the number refers to wattage. Colour temperature may be written 2800 K, or just 28 K. |
HID: high intensity discharge; this class includes the following: M: mercury MH: metal halide S or HS: high pressure sodium Xenon Many elaborate shapes, fixtures. 70–1,000 W+ |
These are very recent additions to museum lighting options; therefore, information in this column is only preliminary. Currently available in many lamp housings, including those used by quartz halogen lamps, e.g. GU10. |
Not Available |
Lifetime, Hours | 10,000 typical | 3,000–40,000+ | 10,000–80,000 (if 70% loss of intensity is failure). | Not Available |
Cost (per lamp) | $10–$40 (varies as size, reflector) | Varies widely with size. | $5–$20, depending on power | Costs "hidden," high: initial building costs, maintenance costs, skylight leaks, energy costs heating/cooling. |
Relamp Cost (per year of 3,000 hr) | $3–$12 | Not Available | ~$0.50–$2.00 assuming 30,000h of current GU10. | Not Available |
Colour Temp (below 3000 K= warm light) (above 4000 K= cool light) |
2700 K warm 3500 K 4100 K cool 5000 K cool |
Mercury, metal halides: warm to cool available. Xenon almost mimics daylight 6500K. |
3000-3500 K available. | Late afternoon: 3000 K Noon sunshine: 6000 K Blue sky: 9000–12,000 K Daylight: mix of above, standard is "D6500 K" |
Colour Rendering Index (CRI) excellent: 90 – 100 good: 80– 89 fair: 70–79 bad: below 70 |
Near 85, good Most compact fluorescent lamps are "triphosphor." their spectrum contains three sharp peaks tuned to our eyes' three colour receptors. They have been unjustifiably criticized simply because they are not smooth spectra. |
Best metal halides are 80 (good) up to 90+(excellent), but most metal halides, mercury, sodium lamps are below 65, bad. | Vary greatly at present. Typically 70 (fair) for all white, 90 (good) expected soon for all white. 90 possible with mixed colour LEDs. | 100, excellent |
UV Output µW/lm | 100–150, medium | Most high to very high. | 0–75, very low. | 300–600 typical, very high |
UV filter Possibilities | Plastic film sleeve or cover must be custom fabricated if necessary. | Many use a glass UV filter against shortwave UV. This does not filter enough UV for museums. Plastic films can be used at some distance from the heat of the bulb. |
Not necessary. |
Window glass filters shortwave UV, but not enough for museums. Laminated glass with middle layer of UV filter available, or self-adhesive plastic films for windows. (Films may void warranty on sealed insulating glass windows.) |
Fibre Optic or Lightpipe Application | Not Available | Small MH or xenon lamps are used in some fibre optic illuminators. Whole rooms of cases have been lit by one powerful lamp in a separate area. This will reduce fire risk, theft risk, and total costs. | Could be used. | Lightpipes have been used for daylight transfer through buildings. |
Main Museum Advantages | Very useful at short distances, such as display cases. Low frequency relamping. Little heat from tube. |
Useful for lighting large areas and museum exteriors for security purposes. | Very useful at short distances, such as display cases. Easily aimed. Very low frequency relamping, low energy use (very economical). No heat in light beam (but lamp itself needs cooling). |
Feels good, looks nice. Can provide very high intensities without high heat content. Can be (but often not) good in terms of sustainability and environment. |
Main Museum Disadvantages | Not easily directed in a sharp beam. | Most have terrible CRI. Most slow to start. Intra-batch variation high. Output can change significantly with ageing. |
Currently CRI and lifetime, highly variable. Colour homogeneity of beam can be poor. Lamp intensity can drop early in lifetime. |
Difficult to control intensity. Varies with the weather, seasons. Windows and their control fittings are expensive to build and to maintain. Can be energy expensive for building operation. |
Colour Rendering Index
Colour Rendering Index (CRI) measures light quality in terms of the viewer's ability to see colours correctly. The scale has a maximum of 100 and no units. CRI is derived by a colourimetric calculation performed on up to 14 different colour samples illuminated by the light source in question, compared to the calculation using daylight or an incandescent lamp as reference. While recognized as imperfect in its correlation with our visual system, CRI is still the best indicator currently available.
There is no international museum standard on what is or is not an "acceptable" CRI, but the Canadian Conservation Institute (CCI) recommends a minimum of 85. Many museums specify greater than 90. That being said, the difference between a compact fluorescent lamp scoring 82, for example, and the guideline 85, is not noticeable by most people in most situations. If such a lamp has major design, cost, and energy advantages, it makes sense to use it. Light sources easily seen as poor, such as the lowest-cost commercial fluorescent lamps, can score below 60.
Note that daylight of CRI 100, after reflecting against a coloured wall or floor, may measure a far worse CRI than light that comes directly from a lamp of CRI 85. If one chooses to illuminate using "bounced" daylight (or any other light source), then the reflector must not be coloured.
Correlated Colour Temperature
Correlated Colour Temperature (CCT) measures the quality of light that passes from "cool" to "warm." This is not a scale of good to bad, unless one is arguing a personal preference for some types of object. The units are degrees Kelvin, abbreviated simply to K. The common terms for this parameter are, unfortunately, contradictory and confusing. A "cool" light source has a high colour temperature and a "warm" light source has a low colour temperature. This comes from our use of the phrase "warm light" to refer to the golden light of sunrise and flames, and "cool light" to refer to the blue skylight that illuminates shaded areas.
With low light levels, as in museums, viewers tend to prefer warmer light similar to that of incandescent lamps, e.g. the 2800 K of standard incandescent lamps, or the slightly higher 3000 K of quartz halogen incandescent lamps. As illumination increases to several thousand lux, preference is for cooler light, 5000 K or higher. The most common energy-efficient lamps (fluorescent lamps) are available in a wide range of colour temperatures. Successful use of compact fluorescent lamps in small museums requires careful attention to colour temperature. Lamps producing warm light, usually marked with 2800 K, or simply "28 K" are generally preferred at lower light levels, as noted earlier. However, lamps producing cooler light (3500 K up to 5000 K) can increase the colour contrast of objects, which may also be desirable. In conclusion, one should always test before making a final choice on colour temperature.
Natural Versus Artificial Light
Proponents of daylight in museums often use the terminological trick of "natural" light to mean daylight, and "artificial" light to mean electric sources, but all light sources are natural, whether glowing stars, glowing filaments, or glowing phosphors. The correct question is whether the CRI is good enough, and, as noted above, both daylight and electric lights can be either good or bad in CRI. The psychological appeal of windows and skylights comes about from the connection to the outside and from the high intensity of the light (when the sun shines). Careful treatment of existing windows by solar screens, blinds, partially closed curtains, and outdoor shutters closed during peak daylight, can reduce the fading and glare risks, while leaving intact the highly desirable visual connection to the outdoors.
Deterioration by Light, UV, and IR
Practical generalizations about deterioration by light, UV, and IR
Given the three distinct bands of radiation — light, ultraviolet, and infrared — one can make useful generalizations about the types of deterioration they cause in museums:
- Light fades (or "bleaches" colours). Those colours that fade can disappear within as little as a few hours of direct sunshine, or just a few years at low museum lighting (e.g. some felt tip pen inks, some colour photographs). Those which do not fade may last centuries in direct sunshine (e.g. ceramics, Minoan frescoes). All coloured objects fall somewhere between these two extremes.
- UV causes yellowing, chalking, weakening, and/or disintegration of materials. Chalking of paint media is often mistaken for pigment fading.
- IR heats the surface of objects, and thus becomes a form of incorrect temperature (too high), with all the damage possibilities outlined in the section on Incorrect temperature. IR will not be considered in any detail in this section.
There is some overlap in the forms of deterioration caused by light and UV. Light (especially violet) can cause some of the disintegration and yellowing listed under UV, but only in a few materials, and only very slowly in comparison to UV. In turn, UV does contribute to the fading of colours, but its contribution becomes dominant only for colours that are durable to light.
None of these overlaps reduces the practical reliability of the above generalizations. To reduce the fading of collections due to display lighting, especially the most rapid fading, there is only one option: reduce light exposure. Many museums, private donors, and their framers have assumed that the primary cause of fading is UV, and that a good UV filter would prevent their collections from fading. Some advertisements for UV filters imply the same. For colours that are sensitive to light — the crux of the museum lighting dilemma — UV usually contributes less than half of the fading and often only one tenth; therefore, it does not allow one to think any differently about reducing light exposure. (The exposure scales in the centre of Table 3 quantify this phenomenon.)
Why bother, then, with UV control? Because for many artifacts, such as paintings with permanent pigments or monochromatic prints and drawings, the yellowing and disintegration of the media and support by UV is the major form of deterioration suffered during uncontrolled museum lighting.
Rates of Light Deterioration
Light damages the colours of some objects — most such colours fade (most of the colours in Figure 3a and 3b) and a few darken (the vermilion in Figure 3a). Table 3 summarizes the available data on the rate of this damage. Coloured materials are divided into four broad categories of sensitivity to light: none; low; medium; and high. For each category, the table provides estimates of the time it takes at various lux levels for the fading to start (first be noticeable) and for it to end (almost no original colour left). One can see that although the range, or uncertainty, within a category is very wide, the differences from one category to the next are much wider. Much of the variation in people's perception of whether light fading is a risk or not arises because this range of sensitivity is so dramatic — some colours in old objects that look fragile can indeed last many centuries, while some colours disappear within our own lifetime, or even in just a few years.
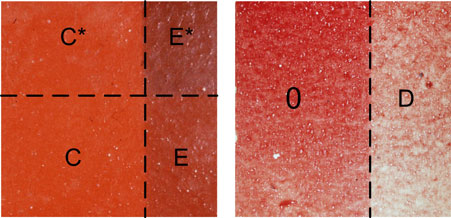
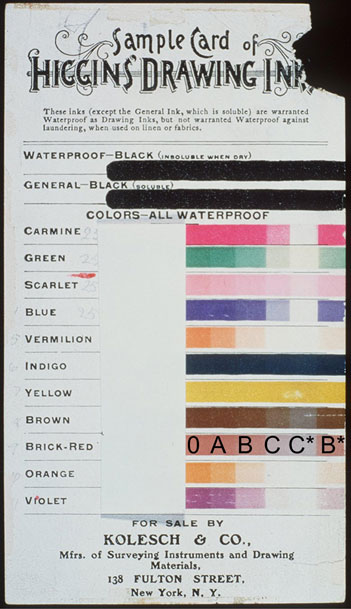
(3a) Oil paints, on the left vermilion darkening and; on the right, carmine lake glaze on white, fading. (3b) Drawing inks on paper, all fading. The letters on the samples indicate the following exposures: 0 - unexposed; A - 0.17 Mlx h; B – 1.7 Mlx h; C – 6.2 Mlx h; D – 17 Mlx h; E – 67 Mlx h. Equivalent exposures range from A: 1 day of sunlight or 1 year at 50 lux to D: 8 months sunlight or 400 years at 50 lux. All areas are protected by a UV filter except areas marked with an asterisk (*). Note that the differences between the presence or absence of a UV filter (B vs. B*, C vs. C*, D vs. D*), while sometimes noticeable, are much less significant than differences between different exposures (A vs. B vs. C vs. D).
Table 3: Sensitivity Levels and Fading
No sensitivity | Low sensitivity | Medium sensitivity | High sensitivity | |
---|---|---|---|---|
Materials that do not change colour due to light. (These materials may change colour due to ageing or pollutants.) Most but not all mineral pigments. |
Materials rated ISO Blue Wool #7, #8 (and higher). Artists palettes classified as "permanent" (a mix of truly permanent AND low-light sensitivity paints, e.g. ASTM D4303 Category I; Winsor and Newton AA). |
Materials rated ISO Blue Wool #4, #5, or #6. Alizarin dyes and lakes. A few historic plant extracts, particularly madder-type reds containing primarily alizarin, as a dye on wool or as a lake pigment in all media. It varies throughout the range of medium and can reach into the low category, depending on concentration, substrate, and mordant. |
Materials rated ISO Blue Wool #1, #2, or #3. Most plant extracts, hence most historic bright dyes and lake pigments in all media: yellows, oranges, greens, purples, many reds, blues. |
|
Exposure Amount | Fade Amount | Time in years for fading | ||
50 lux | Just noticeable fade | 300 – 7000 years | 20 – 700 years | 1.5 – 20 years |
Almost total fade | 10,000 – 200,000 years | 700 – 20,000 years | 50 – 600 years | |
150 lux | Just noticeable fade | 100 – 2,000 years | 7 – 200 years | 1/2 – 7years |
Almost total fade | 3,000 – 70,000 years | 200 – 7,000 years | 15 – 200 years | |
500 lux office | Just noticeable fade | 30 – 700 years | 2 – 70 years | 1/7 – 2 years |
Almost total fade | 1,000 – 20,000 years | 70 – 2,000 years | 5 – 60 years | |
5,000 lux window or study lamp | Just noticeable fade | 3 – 70 years | 2 months – 7 | 5 days – 2 months |
Almost total fade | 100 – 2,000 years | 7 – 200 years | 6 months – 6 years | |
30,000 lux average daylight | Just noticeable fade | 6 months – 10 years | 2 weeks – 1 years | 1 day – 2 weeks |
Almost total fade | 20 – 300 years | 1 – 30 years | 1 month – 1 years | |
Each day of exposure is assumed to be 8 hours, each year 3,000 hours. Time for a "just noticeable fade" is given as a range based on the doses for the range of ISO Blue Wools in that sensitivity category (consult Table 4). The "almost total fade" is based on a conservative estimate of 30× the "just noticeable fade," although fading often slows down, so that an estimate of 100×, the just noticeable fade is probable for many colours. |
The broad sensitivity categories of Table 3 (high, medium, and low) were adopted in a recent international guideline for museum lighting (CIE ). They are defined using the industrial lightfastness standards known as the ISO Blue Wools. These are a set of textiles, originally numbered #1 to #8, each about 2 to 3 times as sensitive as the next. High sensitivity was defined as materials rated #1, #2, or #3; medium as #4, #5, or #6; and low as #7, #8, or higher (more were added to the original eight Blue Wools as needed by industry). The Blue Wool numbers are the main route into the literature on colourant sensitivity, as reviewed in Michalski ( and ) and as summarized in a more detailed version of Table 3 contained in the CIE guideline ().
The conversion of a Blue Wool rating into an estimate of the light exposure that will cause just noticeable fading is provided in Table 4, derived from a review of the literature partially described in Michalski (). The estimates in Table 4 are the basis of the time to fade estimates in Table 3.
The Blue Wools as an estimate of the range of sensitivities in collections
Museums inevitably ask, what is the range of colourant sensitivities in my collection? The original eight Blue Wools, developed in the 1920s, represented the sensitivity range that the dye and colourant industry knew was reflected in all the coloured goods of the time, whether using natural dyes, synthetic dyes (started in the 19th century), or even pigments. Thus the range of these eight Blue Wools is an excellent estimate of the range of light-fading sensitivities one might expect to find in a mixed museum collection. Of course, some coloured objects are not sensitive at all. Some coloured objects are even more sensitive than #1 because they were not intended to last even as long as a poor-quality textile, e.g. some felt tip pens.
Detail | Light dose (Mlx h) to cause a "just noticeable fade" of the Blue Wool standards | |||||||
---|---|---|---|---|---|---|---|---|
ISO Blue Wool number #8 | ISO Blue Wool number #7 | ISO Blue Wool number #6 | ISO Blue Wool number #5 | ISO Blue Wool number #4 | ISO Blue Wool number #3 | ISO Blue Wool number #2 | ISO Blue Wool number #1 | |
Dose for "just noticeable fade" if UV present | 120 | 50 | 20 | 8 | 3.5 | 1.5 | 0.6 | 0.22 |
Dose for "just noticeable fade" if UV removed | 1000 | 300 | 100 | 30 | 10 | 3 | 1 | 0.3 |
Sensitivity category used in Table 3 | Low sensitivity | Medium sensitivity | High sensitivity |
Rates of UV Deterioration
The disintegration of organic materials caused by UV takes many forms, such as the weakening of textile fibres, the weathering of wood and bone, and the chalking of paints shown in Figure 4. Yellowing caused by UV is most easily seen in poor-quality plastic and paper such as newsprint. Table 5 summarizes the various effects and rates of damage known for UV. It begins with the benchmark of what we know from outdoor daylight exposure studies and extrapolates to the lesser UV exposures due to filtration by glass and by UV filters.

The images are all for an area exposed to 67 Mlx h of a light source similar to daylight through a window (equivalent to about 8 months full daylight, or 400 years of display at 50 lux). On the left is an optical microscope view. The bottom half was protected by a good UV filter. The black-and-white images to the right are scanning electron micrographs of the top and bottom areas. The lower image shows the smooth oil medium surface undamaged by UV and the upper image shows the eroded and cracked surface damaged by UV. The brown (mineral) pigment is not affected by either light or UV.
No sensitivity | Low sensitivity | Medium sensitivity | High sensitivity | Very high sensitivity | |
---|---|---|---|---|---|
Inorganic materials: metals, stone, ceramics, glass. |
Cracking, chalking of modern plastics, rubbers, paints that contain UV stabilizers, designed for outdoor exposure. |
Wood turns grey, erodes. |
Chalking of oil paints with photosensitizing pigments (zinc white, early titanium white). |
Yellowing of some low quality papers, such as newsprint. | |
Daylight | Lux Amount | Approximate time to cause damage described above | |||
Daylight spectrum ~600-1000 µW/lm | Daily outdoor average: 30,000 lux | ~10 years | ~1 years (wood erosion: 50µm of surface per year) | ~1 month | ~3 days |
50 lux | ~5,000 years (thermal ageingTable Footnote 1 likely in 100–1,000 years at 20°C) |
~500 years (thermal ageingTable Footnote 1 likely in 100–1,000 years at 20°C) |
~50 years | ~5 years | |
Daylight through window glass, ~400-500 µW/lm | Full daylight 30,000 lux |
~30 years or moreTable Footnote 2 (thermal ageingTable Footnote 1 likely in 5–50 years at 40°C)Table Footnote 3 |
~3 years or moreTable Footnote 2 (thermal ageingTable Footnote 1 likely in 5–50 years at 40°C)Table Footnote 3 |
~2 months or moreTable Footnote 2 | ~1 month or moreTable Footnote 2 (thermal ageingTable Footnote 1 likely in 2 years at 40°C)Table Footnote 3 |
50 lux | ~20,000 years or moreTable Footnote 2 (thermal ageingTable Footnote 1 likely in 100–1,000 years at 20°C) |
~2,000 years or moreTable Footnote 2 (thermal ageingTable Footnote 1 likely in 100–1,000 years at 20°C) |
~100 years or moreTable Footnote 2 (thermal ageingTable Footnote 1 likely in 100–1,000 years at 20°C) |
~50 years or moreTable Footnote 2 (thermal ageingTable Footnote 1 likely by ~30 years at 20°C) |
|
Daylight with good UV filter ~75 µW/lm or less | Full daylight 30,000 lux |
~300 years or moreTable Footnote 2 (thermal ageingTable Footnote 1 likely in 100–1,000 years at 20°C) |
~30 years or moreTable Footnote 2 (thermal ageingTable Footnote 1 likely in 5–50 years at 40°C)Table Footnote 3 |
~2 years or moreTable Footnote 2 (thermal ageingTable Footnote 1 likely in 5–50 years at 40°C)Table Footnote 3 |
Bleaching by blue light overrides any residual UV yellowing (thermal yellowing may eventually prevail) |
50 lux | ~many millennia (thermal ageingTable Footnote 1 likely in 100–1,000 years at 20°C) |
~many millennia (thermal ageingTable Footnote 1 likely in 100–1,000 years at 20°C) |
~many millennia (thermal ageingTable Footnote 1 likely in 100–1,000 years at 20°C) |
Note in Table 5 the strong role of reduced light intensity, not just the degree of UV filtration, in extending the lifetime of materials on display. When UV is measured as a ratio — microwatts (of UV energy) per lumen (of light) — then the total UV exposure, and the damage that depends on it, is proportional to both the intensity of the lighting and the UV measurement.
Rates of IR Deterioration
Infrared causes heating (considered in detail in the section Incorrect temperature). IR heating usually becomes a problem only with two sources of light: incandescent lamps at high intensity, over 5000 lux, and direct sunlight. In Table 5, nominally about UV damage, the effects of elevated object temperatures by direct sunlight are noted in the rows for average daylight through window glass. Sunlight, or intense incandescent lighting, can warm surfaces 40°C above ambient, or more. This elevates the rate of thermal decay by a factor of 20 or more.
When Light, UV, IR, and Other Agents of Deterioration Mix in the Same Object
Different deterioration phenomena often occur simultaneously: the yellowing or weakening caused by UV can be mixed with similar effects caused by thermal ageing. This thermal ageing is, in turn, accelerated by the high temperatures possible with IR (as noted in Table 5). On top of that, some of the new yellowing may be faded by light (blue light in particular). All of us have seen old framed prints with various patterns of yellowing. These provide an interesting amalgam of agent effects. To begin with, any coloured paints and inks may be faded by light. The paper may be yellowed by UV that the glass does not block, but under the matt, it will be protected. In extreme exposures, the fibres of the paper weaken (often not noticed until such prints are handled or washed during conservation treatment and the image area begins to disintegrate). If the matt is of poor quality, it will emit vapours that cause a narrow band of yellow/brown near the edge of the matt, which is greatly accelerated by IR heating. If a good UV filter is present, the paper in the image area will become whiter, not yellow, but the region under the matt will become uniformly yellow from thermal yellowing, which is accelerated by IR heating. The permutations may be complex, but the conclusions are simple: for organic materials, keeping the light intensity below many thousands of lux will reduce all forms of light, UV, and IR damage. In addition, using low UV light sources will push UV damage of high-sensitivity materials well below the similar forms of damage caused by room temperature itself.
Control of Light, UV, and IR
Stages of Control
Avoid
- Establish rules for light levels, UV levels, and light sources (see the "Control strategies" section below).
- Bring outdoor objects indoors.
- Switch off electric lights whenever no viewer is present. Use proximity switches whenever possible.
- In historic houses, select locations in the house, and within the room, that are low in light intensity throughout the day. If there are no UV filters on the windows, place objects where no direct light from the window can reach them.
Block
- Use UV filters on light sources that are high in UV (as indicated in Table 2).
- Outdoors, use shading devices such as simple roofs or take advantage of the north side of a building.
- Indoors, use screens, louvers, blinds, solar screen, paint, etc. to block windows.
- Separate bright public access areas from display areas and provide adaptation paths between the two.
- Close curtains, blinds, shutters, etc. when the museum is closed. Cover cases when no viewers are present.
Detect
- Look for signs of light and UV damage in the museum.
- Use light meters and passive dosimeters.
- Use museum UV meters.
- Use a simple thermometer, if an IR heating problem is suspected.
Respond
- When faded objects are noted, determine causes and possible solutions.
- When light meters and UV meters show unexpectedly high values in a location, determine causes and solutions.
Recover
- There is no true recovery possible from faded colours or disintegrated surfaces. Restoring such losses requires replacement by new material.
Control Strategies for Different Degrees of Preservation
Introduction to different degrees of preservation
We can all agree on the general museum goal of reducing light damage at the same time as giving visual access, but, in practical terms, how much of each becomes a matter of increasing difficulty. There are three strategies, increasingly effective, but increasingly difficult:
- Follow a few basic measures designed to eliminate the extremes of light exposure.
- Follow a simple rule based on the light intensity for minimum visibility, 50 lux.
- Follow a few difficult rules to both minimize damage and maximize visibility.
A basic strategy for small museums: Eliminate all extreme light exposures
From the List of Basics introduced in section I, those that influence light and UV:
- A reliable roof that covers all organic artifacts (and preferably most inorganic artifacts). While this is obvious to even people outside museums, it also applies to large objects, such as historic vehicles or historic machines with paint. They cannot be expected to survive many years if exposed to sun and weather.
- Reliable walls, windows, and doors that block local weather, sunlight, local pests, amateur thieves, and vandals.
- Avoid areas of direct sunlight and intense spot lamps at close distances on all organic artifacts.
Results from the basic strategy
Assuming these measures avoid the extremes of 30,000 lux of average daylight, and fall somewhere between the 5000 lux of windows and the 500 lux of most office lighting, then low-sensitivity objects on display for a century will still appear colourful. Medium-sensitivity objects will have faded already in little more than a decade, and high-sensitivity objects will have been destroyed long ago, unless they were serendipitously set aside in dark forgotten places, boxes, envelopes, dowry chests, bound in volumes, etc., or were recently acquired from such places to be put on museum display. This is the tragedy of small historic house museums that acquire colourful new treasures from donors who had kept them in dark storage.
The traditional rule-driven strategy: Light everything at a fixed, low intensity
The traditional museum lighting rules, as contained in various publications of the s and s including CCI's own Technical Bulletins, were based on the 50 lux benchmark and added two extra categories for presumed differences in sensitivity:
- 50 lux for textiles, works on paper, watercolours on any medium, photographs, feathers, etc.;
- 150 lux for all oil and acrylic paint surfaces, polychrome, panels, furniture, etc.; and
- 300 lux for stone, metal, etc., primarily to avoid contrasting lighting.
Different authors' lists tended to differ somewhat regarding which items were in which category, and whether to include the 300 lux category.
The traditional rule on UV was as follows:
- Keep all UV levels below 75 µW/lm (the value for ordinary incandescent lamps).
The maximum acceptable UV level was established in the s, based on the UV emitted by ordinary incandescent lamps. Experience indicated that these light sources provoked very little if any UV damage on mixed historic collections over many decades, given low-light intensities.
Furthermore, common practice tended to add these rules as well:
- Exposure times were defined primarily by operational considerations.
- Objects with components of many different sensitivities were defined by their weakest component.
Results from the traditional rule-driven strategy
The traditional lighting policy underlies most current lending and borrowing requirements. It reduces damage across all collections (as compared to normal building light levels), but high-sensitivity artifacts will still fade significantly within a few decades and low-sensitivity artifacts will be difficult to see for no good reason (except the simplicity of simple rules). If objects are dark, of low contrast, or highly detailed, they will, in fact, be impossible for many to see meaningfully at all. The presumed difference in sensitivity of the two categories — paper and textiles versus paintings and polychrome — is not warranted. While one might argue that the average watercolour is more sensitive than the average oil painting (due to the preponderance of thin washes), the fact remains that one can find large and important groups of contrary examples. All portraits in oil of the last several centuries depend on red lakes of high-to-medium sensitivity. When these fade (as many have already), the colour of the skin of the subject changes from the original, rosy "living" colour to a "dead" white colour. Conversely, whole classes of paper objects have been made with low or even zero sensitivity colourants, such as carbon blacks, ochres, white chalks, etc.
A risk-management strategy: Accept and manage fading and visibility
A detailed lighting policy within a larger risk-management framework acknowledges explicitly that colourants fade and that visibility improves with more light, and then develops a policy based on the following steps:
- Establish a criterion for an acceptable rate of fading (acceptable risk). This is usually expressed as the time period that causes just noticeable fading. This might be selected as 100 years, or 30 years, or 300, etc.
- Assess sensitivities. At the moment, this tends to large generalizations not unlike the groupings of the traditional rules above, such as "watercolours," but it can incorporate more detailed assessments, such as important sub-groups, a certain genre, or even a particular object of great value, using information such as in Table 3. Generally, the highest sensitivity colourant found, or expected, characterizes the whole group.
- Consider visibility. Begin by assuming the 50 lux benchmark, but if a collection does not contain any high- or even medium-sensitivity colourants, one can consider adjusting lux levels upwards, based on Table 1. One can also consider mixing short periods of better visual access with long periods of minimal visual access, especially to accommodate older viewers, or special inspections by scholars.
- Consider the lux levels practically available, given the lighting equipment.
- Determine display time. This is the inevitable result of calculating what display rotation will keep fading within the acceptable fading criterion set at the start. For example, from Table 3, the shortest time required to reach a just noticeable fade of the high-sensitivity category is 1.5 years; therefore, high-sensitivity colourants can only be on display about 1.5% of the time, given the 100 year criterion set at the beginning.
At present, policies following similar steps have been described by the Montreal Museum of Fine Arts (Colby ) and the Victoria and Albert Museum (Ashley-Smith et al. ).
In small museums and historic houses, where little or no light control exists, the steps vary slightly:
- As above, recognizing that perhaps the museum mandate for preservation is not the same as that of a national museum.
- As above, recognizing that a smaller museum can often get to know its collection better than a national museum.
- As above, recognizing that on the one hand, visitors to a smaller community museum may be older on average and, on the other, that visitors may expect less visibility in a historic house setting.
- Assess the light intensities, or cumulative exposures, in different display areas.
- Determine display time possible in the locations under consideration for the artifact, given 1, 2, and 4. Balance where something is displayed, and how long it can be displayed there, or change your criterion in 1.
Optimum strategy: Results
The museum will manage explicitly the lifetime of the colours in its collections, at the same time as increasing the visibility of the many objects on display that are low or even zero sensitivity. This strategy requires considerable investment of expert knowledge and will provoke custodial anxiety due to uncertainty. For example, exactly how low is the sensitivity of a black-and-white photograph or carbon ink lithograph – at least hundreds, possibly thousands, of times lower than the average colour photograph or chromolithograph, and much more likely to be damaged by pollutants or thermal ageing before being affected by permanent display at 500 lux (using good UV filters). It also requires considerable labour to assess large collections. In current practice, this method will probably be used only to augment the simple rule-driven strategy — such as developing exhibition policies of reduced exposure time for high-sensitivity materials and examining explicitly the display of any especially valuable artifacts. Widespread use of this method will only become possible with the gradual accumulation and dissemination of the sensitivity distributions of useful categories, such as the palette of a particular artist's works, the costumes of a particular period, the photographs of a particular manufacturer, etc.
To help make such decisions using a risk management strategy, CCI has developed a light damage calculator for the web. It allows one to explore quickly the likely fading of different objects under a wide range of lux levels and display schedules. As sensitivity data is provided by researchers worldwide, it will be made available through this web page.
Conclusions
"Seeing versus saving" is the epitome of the "use versus preservation" dilemma faced by museums. We depend on light for sight, but the fading is utterly irreversible. In the past, museums relied on a simple rule derived from the "adequate" visibility of 50 lux. Loan agreements and governmental guidelines still reflect that rule. Museums with full control of their lighting behaved as if conformity to such rules meant the fading risk had vanished. Perhaps they had reached the point where their anxiety had been allayed by a workable compromise that could be codified. Small museums with less ability to control lighting were given no guidance on which objects were truly at risk from intense light and which were not. Moving beyond a rules-based or fatalistic approach, towards a strategy of risk assessment (aided by the CCI light damage calculator) smaller museums will be able to focus their efforts, to place artifacts strategically within the varied light levels of their rooms, and to relax where the fading risk is small or non-existent.
Vignettes
Vignette 1. Use of a Window Well in a Historic House for Display
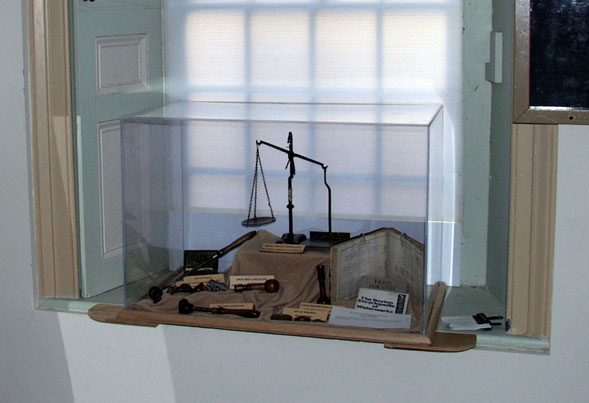
Here, two strategies have been taken: (1) The window has been screened by a translucent fluted plastic board, usually used for graphic signs. This reduces the light intensity by about one half (it also adds to the insulating quality of the window). This is a simple method using a few pushpins, easily removed if the room use changes. Although the glare from the translucent panel is less than ideal, the background fabric immediately adjacent to the objects is dark and matte. (2) Most importantly, zero sensitivity objects (metal stamps) have been selected for this display, or low-sensitivity artifacts (white paper, black ink, unstained wood). The brass stamps are very detailed, dark, low-contrast objects; therefore, seeing them well takes advantage of the strong window light.
Vignette 2: A Local Art Gallery with Basic Track Lighting
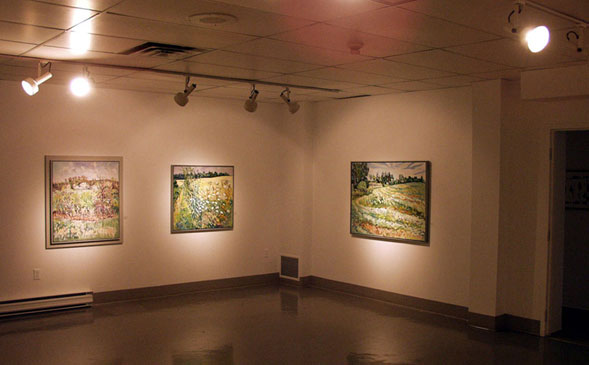
Unlike the historic house with its original window wells described in the earlier vignette, a purpose-built display area, such as this small gallery at the Peel Regional Art Gallery, Brampton, Ontario, has full control of the lighting, both ambient and on the artworks. It uses a basic track pattern, one strip along each of the long walls, about 1.5 m from the wall, so that the light beam hits the painting centre at approximately 30° (to the vertical). The end walls are lit from the last portion of track. Note that glare control by the full lamp housings is very good when facing away from the viewer, but less successful when trying to light the left end wall. The spot lamps place the emphasis on the paintings and reduce competition from the walls, but finding spot lamps that yield moderate intensities at close quarters is difficult. Without knowing the artists' palettes, the gallery must assume some high-sensitivity colourants are in use. Because many of the artists selected for this gallery are local and alive, the museum could ask for information on the palettes, obtain their sensitivities, or even advise current artists about low-sensitivity palettes.
References
-
Colby, Karen M. "A Suggested Exhibition Policy for Works of Art on Paper. Journal of the International Institute for Conservation-Canadian Group. 17 (), pp. 3-11.
-
Michalski, S. "Damage to Museum Objects by Visible Radiation (Light) and Ultraviolet Radiation (UV)." In, Lighting in Museums, Galleries and Historic Houses. London: Museums Association, UKIC, and Group of Designers and Interpreters for Museums, , pp. 3-16.
Key Readings
-
Commission internationale de l'éclairage (CIE). Control of Damage to Museum Objects by Optical Radiation. CIE Technical Report, 157. Vienna: Commission internationale de l'éclairage, .
-
Ashley-Smith, J., A. Derbyshire, and B. Pretzel. "The Continuing Development of a Practical Lighting Policy for Works of Art on Paper and Other Object Types at the Victoria and Albert Museum." ICOM Committee for Conservation. In, Triennial meeting (13th), Rio de Janeiro, -: Preprints. London: James & James, , p. 3-8.
-
Michalski, S. "The Lighting Decision." In, Fabric of an Exhibition, Preprints of Textile Symposium 97. Ottawa: Canadian Conservation Institute, , pp. 97-104.
Glossary
- Blue Wool Scale:
- A scale for sensitivity to light fading, based on a set of eight different dyed pieces of wool.
- Foot candle:
- The Imperial unit of illuminance (light intensity) equal to one lumen per square foot or 10.76 lux.
- Just noticeable fade:
- "Just noticeable" varies with observers, situations, and industrial conventions, but for practical purposes, it means more or less just what it says. Technically, it is defined here using the ISO convention of "GS4," the first full step on the scale of five paired grey squares used to measure fading during lightfastness testing. In other words, it is the change in colour that the industry considered represented "just noticeable" from a practical user's perspective. In colourimetric units, GS4 represents ΔE=1.8. "Just noticeable" here must not be confused with the "just perceptible colour difference" that humans can see during optimal viewing circumstances, which is 2 to 6 times smaller than ΔE=1.8, depending on colour. (Colorimetic systems such as ΔE (CIELAB) are an attempt, currently far from perfect, to find a metric that will have as its unit the "just perceptible difference" across the whole colour space.)
- Lumen:
- The SI unit of luminous flux (light) used to rate the light output of lamps in manufacturer's catalogues.
- Lux:
- The SI (metric) unit of illuminance (light intensity) defined as 1 lumen per square meter. Direct noon sunlight is almost 100,000 lux; 1 lux is about the intensity of light from a candle at 1 m (photometric units were originally defined literally in terms of a "standard candle" at one metre).
- Mlx h:
- Abbreviation of megalux-hour. A museum unit of light exposure, or light dose. Equal to the product of light intensity (lux) and time (hours), quantified in millions of lux hours. The use of the time unit hours is incorrect within SI rules, but this particular usage is common in museum conservation literature.
- µW/lm:
- Abbreviation of microwatts per lumen. The museum unit of UV radiation. It is the ratio of UV intensity (in SI radiometric units µW/m2) to light intensity (in SI photometric units, lux= lumen/m2), hence the result µW/lm.
Thanks to the Centro Nacional de Conservación y Restauración in Chile, the Canadian Conservation Institute’s web resource Agents of deterioration, translated into Spanish by ICCROM, is now available free of charge. Intended for curators and conservators, the resource identifies 10 primary threats specific to heritage environments.
Page details
- Date modified: