Agent of Deterioration: Physical Forces
Paul Marcon
Force Definitions
Physical force can damage objects directly by causing rotation, deformation, stress, and pressure. It may also damage objects indirectly by causing collision between objects or object parts. Damage from physical force ranges from imperceptible hairline fissures and minute losses, to large-scale effects such as crushing objects, collapsing floors, and, in extreme cases, destroying buildings. Five important force-related effects are: impact; shock; vibration; pressure; and abrasion. Some of these effects are closely linked. They are defined as follows:
Impact is the result of something striking an object, an object striking a hard surface, or objects striking each other. Impact force can be concentrated in a small area or spread out due to the hardness and geometry of the surfaces that collide. Localized impact damage, such as small cracks, can increase the susceptibility of an object to force.
Shock is usually the result of a strong impact. It can induce large deformations and strains in objects or their parts. Shock intensity is measured in g units of acceleration where one g represents the acceleration due to the Earth's gravity. For example, if an object is subjected to a shock of 100 g, the object will sustain a force equal to 100 times its weight for a brief (several to 10 or more milliseconds) period of time. Shock can cause substantial damage to most art objects and is an important cause of damage during shipment.
Vibration is the oscillating motion of an object relative to a fixed point of reference. A vibration-prone object will vibrate freely (free vibration) if it is displaced from its equilibrium position and released. A tuning fork provides an example of this; it vibrates freely at one frequency after it is struck. The vibration amplitude then diminishes over time as the energy that was supplied to the tuning fork is released (Figure 1a). An object may also vibrate in response to an externally applied source of vibration (forced vibration) as in the case of a packaged item inside a transport vehicle. The simplest form of continuous vibration is harmonic motion, which consists of movement that repeats itself exactly after certain time periods, as illustrated in Figure 1b. Random vibration is a type of vibration found in moving vehicles and other everyday vibration sources. It is a complex combination of many vibration frequencies with randomly varying amplitudes. Figure 1c shows the appearance of random vibration on a trace of amplitude versus time.
Two basic quantities for describing vibration are frequency (in cycles per second or Hertz [Hz]) and amplitude (which can be expressed as a displacement, velocity, or acceleration). Construction vibration amplitude is expressed as particle velocity (the velocity of a particle as it transmits a wave). This measure correlates well with the development of cosmetic cracking in structures (consult the "Construction Vibration and its Effect on Buildings" section below).
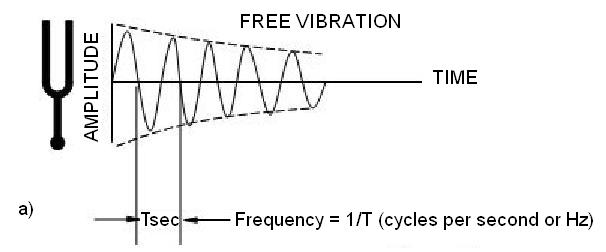
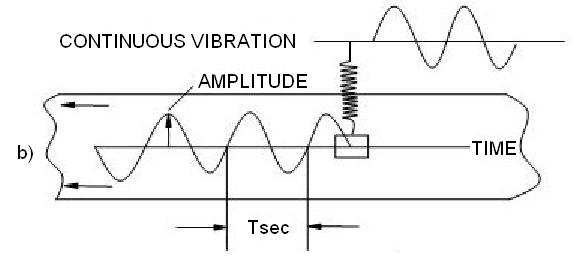
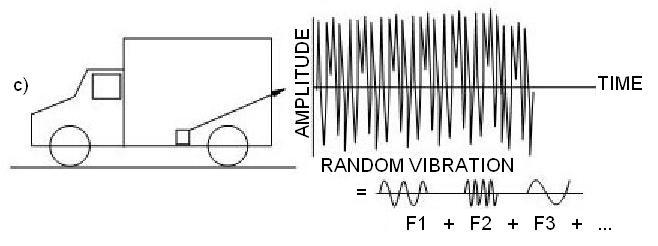
Pressure is the force applied on a unit area of material. Pressure may be the result of gravity or handling. It may contribute to abrasion, strain, and deformation ultimately leading to distortion or breakage. When objects or packages are stacked in storage, compressive loads are applied to the lower items of the stack. Loads on stacked items in transport will be magnified by the shock and vibration caused in moving vehicles. Shipping containers can be designed to carry these compressive loads; the ability to support a top load of 50 pounds per square foot is a common requirement for industrial crating. Pressure (or compressive load) on a flat surface can be simply calculated as force divided by the area on which the force acts. Maximizing the contact area will minimize the load per unit area — an important consideration for fragile surfaces and container top loads (e.g. skids are better at distributing the weight of an upper container onto lower containers of a stack compared to individual feet, which may concentrate the loads onto smaller portions of the supporting container).
Abrasion is possible wherever there is movement between two surfaces that are in contact. Abrasion effects will vary according to surface durability, the amount of pressure applied to the surfaces, and the profile of these surfaces. The presence of abrasive material or particles between surfaces may also cause, or accelerate, abrasion. Abrasive damage may appear after a long period of exposure to movement, but it can also occur quickly if the surface is fragile.
Direct Effects of Force
Force that is directly applied to an object may result incompression, punctures, dents, tears, cracks, chips, scratches, or abrasion. Gravity results in a continuous load on all objects. Substantial loads can be concentrated on object parts during handling operations (consult Figure 2). Improperly made supports may lead to distortion or permanent deformation of objects due to the effects of load concentration. Long-term loading of or short-term overloading of cushioning material results in looseness between the object and the package material, which can reduce the effectiveness of cushioning. Excessive loading of support materials, such as foam materials in storage, may lead to instability of objects. An incident in which a heavy object fell off its support, that had deformed under an excessive load, has been reported. Another incident involved a display plinth under a large marble sculpture that failed after a short period of time. The proper support for heavy items is clearly an important safety issue for staff and visitors to museums.
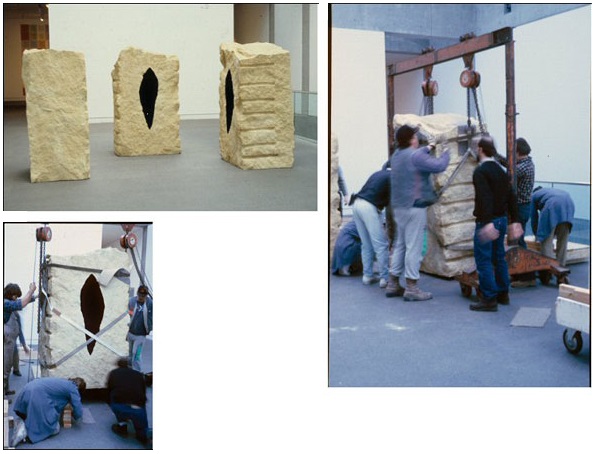
Mechanical Shock
Mechanical shock is an energetic response of an object. It is characterized by substantial displacements and strains. Four outcomes are possible.
- Low levels of shock may be absorbed and dissipated in the object without damage. A bell provides an example: striking it with the right object and the proper amount of force makes it ring without any damage to the bell's surface or structure.
- Impact may cause an object or its parts to move, resulting in collision between objects, object parts, and their surroundings.
- High shock levels may cause movement and induce strains in excess of critical thresholds resulting in fatigue damage (explained below under the heading of vibration).
- If the shock magnitude is high enough, damage occurs in a single event (stress fracture).
Vibration Effects on People
The full range of human perception of, and reactions to, vibration is summarized as a basis for comparison to commonly encountered vibration sources. Humans can perceive vibration at very low amplitudes in the range of 0.1 to 0.5 mm/s. Peak human vibration sensitivity occurs in the frequency range of 5 to 30 Hz, which is the same frequency range that is generated by many construction activities (Dowding ). This explains why concerns and complaints arise during construction work even though the vibration amplitudes generated by this activity may be relatively low.
Vibration Effects on Objects
Most objects are capable of vibration at many vibration frequencies due to their geometry, mass, and elasticity. The lowest vibration frequency is referred to as the natural frequency. Vibration tendencies at higher frequencies are called resonant frequencies. It is also common to refer to all these frequencies as resonant frequencies. Some examples of vibration-prone objects are illustrated in Figure 4.
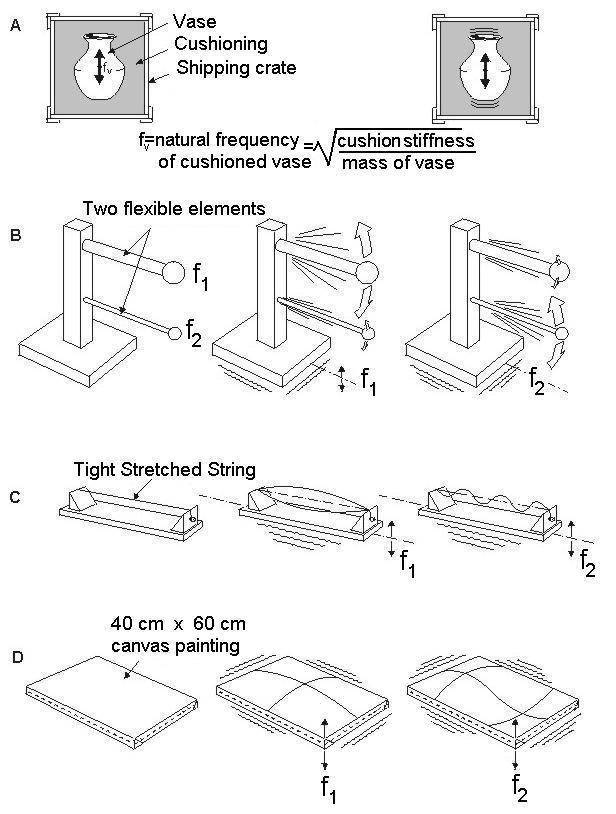
If the package containing the cushioned vase in Figure 5 is subjected to vibration, three outcomes are possible. These three conditions are described below in detail.
Transmission (Figure 5a). If the vibration frequency is lower than fv (f< fv), the vase will vibrate at the same frequency and amplitude as the vibration source.
Resonance (Figure 5b). If the frequency of the vibration source matches the resonant frequency of the vase–cushion combination, fv, the amplitude of the vase will be greater than the amplitude of the vibration source due to a condition known as resonance. Resonance in a cushioning system is normal. Resonant amplitude is limited by the damping properties of most cushioning materials. Package designers usually avoid having object resonances in the same range as the cushion system. Resonance is an important consideration in structures and machinery due to the force-multiplying effects of this condition.
Attenuation (Figure 5c). If the vibration frequency is greater than fv, attenuation takes place and the vase will appear almost motionless while the package oscillates. This is vibration isolation where frequencies greater than 2 x fv will be isolated with an efficiency of 80% or more.
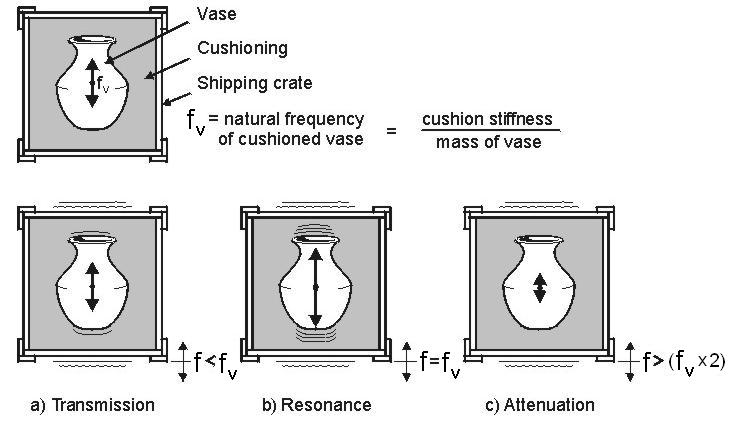
The vase in the preceding example is essentially a rigid object; therefore, none of the vibration conditions described above are hazardous as long as the surface of the vase is durable.
Fatigue
A familiar damaging effect of vibration is mechanical fatigue. In order for this to take place, two requirements must be met. First, a critical stress threshold must be attained or exceeded during each vibration cycle. Damage then occurs after a time interval that depends on the number of vibration cycles and their peak values. The Tacoma Narrows Bridge (consult Figure 6) provides a dramatic example of this effect on a very large scale. Note that if stress levels are below a critical level, then almost indefinite cycling is possible without damage. Stress fracture on the other hand occurs in one cycle of sufficiently high stress.
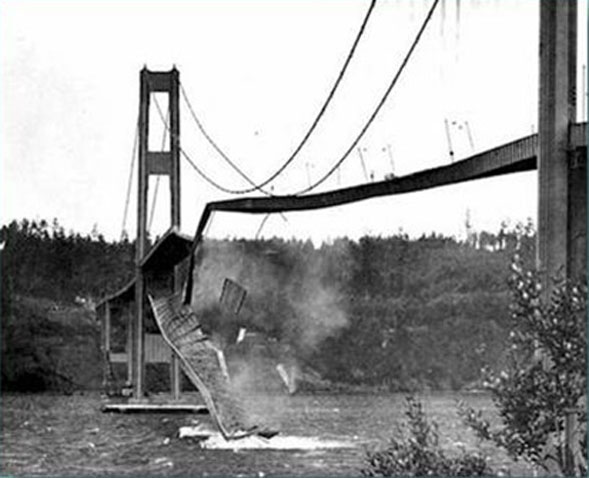
While fatigue is an important issue for machinery and engineering structures, it may not be as important for museum objects in light of the low magnitude of vibration sources encountered in common situations. In experimental investigations using specially prepared canvas paintings and other small-to-medium-size objects, attempts to induce damage by vibration have usually required unrealistically high (compared to commonly encountered sources) vibration levels. The canvas painting in Figure 7 was vibrated for several days at high amplitude, but did not show clear evidence of damage when analyzed using a laser scanner. While it is not possible to apply the results of these investigations to all object classes, the results appear to support the following generalizations:
- There is not always an efficient physical connection between the object and a vibration source to effectively transmit the vibration.
- Practical sources of random vibration encountered by objects do not usually cause the sustained or energetic object responses that result in fatigue.
- Fatigue-like damage (cracks) may already be present in many objects and may be caused by the action of direct forces or other agents such as temperature and humidity cycling.
- If fatigue-type damage already exists, it would be reasonable to expect that additional cycling may contribute to additional damage in some cases.
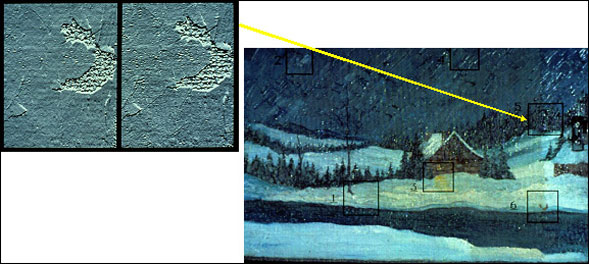
Construction Vibration and its Effects on Buildings
Damage to buildings by construction vibration appears in a form that is described as "cosmetic cracking" in the literature. It is interesting to note that this type of damage is an ongoing process for most buildings, even those located in areas free of vibration, and that temperature and humidity fluctuations (not noticed by the occupants) are important causes of this effect. The findings of 16 building damage studies from Dowding () are summarized in Table 4, below.
Low-level Vibration Effects
Building occupants will react in situations when building vibration is perceptible or where there is some evidence of movement in object parts. Questions about possible risks to collections are often raised in these situations. One involved concerns about visible canvas movement in a collections storage room due to low-level vibration from HVAC system system components. The second involved concerns about perceptible blast-induced vibration from a rock quarry near the site and from nearby road traffic. A brick re-pointing procedure generated clearly perceptible vibration at another client's site where a fragile, hollow plaster exhibit was on loan. Neither vibration scenario was deemed to present a significant risk to the collections. While it is still advisable to investigate concerns carefully, some general opinions on the subject of low-level vibration are provided below:
- Perceptible levels of vibration from low-level sources at a reasonable distance from the vibration-producing activity may not require any corrective action. As the distance from the source to the object increases, the vibration intensity diminishes quickly as it crosses structural connections and is transmitted through building materials.
- Relocation of objects near construction operations is advisable provided that the objects can be moved without an even greater risk of damage due to handling.
- If relocation is not feasible, and the object is in contact with a surface that transmits vibration (e.g. a common floor slab), the object may be decoupled from the floor with a layer of soft cushioning material.
- Object movement (sliding) may be a concern for lighter objects weighing less than 1 kg stored or displayed on a slippery surface near internally generated sources of vibration. These objects can benefit from an interleaf or a method of restraint appropriate for the type, material, and configuration of the object such as wax or an interior restraining block.
Vibration and Sensitive Equipment
Research and conservation activities in museums require the use of vibration-sensitive instruments such as microscopes and precision balances (Figure 8). New building construction with long open spans between support columns and beams bridged by light construction materials will also contribute to vibration-related problems for this type of equipment. Long extension arms on microscopes can re-amplify low-level building vibrations in the instrument itself. Vibration criteria for sensitive equipment are published in standards such as those published by the International Standards Organization (ISO) and by others (). Note that some instruments, such as scanning electron microscopes, will be adversely affected at levels that are far below those that building occupants can perceive.
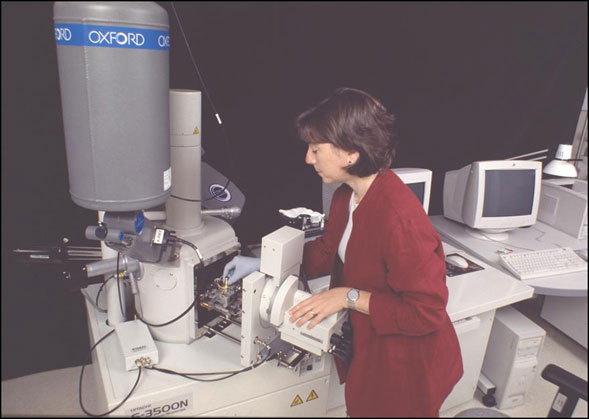
Acoustic Effects
Occasionally, concerns are expressed about the effect of loud music on artwork. The classic case of a shattering wine glass on exposure to loud sound illustrates the structural vulnerability that is required to achieve this effect as well as the precise control and intensity of the sound source that would unlikely be duplicated in anything other than a lab setting. The sound intensity required to break fragile stemware (very fine crystal) is reported to be about 140 decibels (dB) in the direct vicinity of the glass. In addition to high sound intensity, breaking the glass also requires identifying a critical resonant of the glass precisely and adjusting the high-intensity vibration source to within 0.5 Hz of this frequency.
Attempts to develop non-destructive analysis methods of sample frescos and icons to detect interlayer delamination and other forms of damage required sound pressure levels of 80 to 110 dB at the fresco surface (Castellini ). Loudspeakers were ineffective in inducing sufficient movement in the fresco components to enable their analysis by scanning laser methods. Horns and parabolic mirrors and direct attachment of transducers were necessary in order to apply sufficient sound pressures to the areas under investigation. It is interesting to note that the goal of this analysis method was:
- to evaluate the condition of the frescoes non-destructively; and
- to seek an alternative for an expensive and time consuming manual investigation method that involves tapping small areas and sensing any vibration with their fingertips while listening to the induced sound.
This manual technique is reported to impose a peak pressure of about 30 N/cm2 on the sampled area. Horns and speakers impose about 6.5E-04 N/cm2 and a direct transducer attachment imposes about 0.5 N/cm2. The manual investigation method also shares the goal of non-destructive condition assessment and applies force intensities to the sampled item that are about 50,000 times greater than high-intensity acoustic sources and 230,000 times greater than the force intensity imposed on the fresco samples by live music.
In summary, acoustic sources such as live music do not appear to pose a significant risk to artwork. Ordinary handling, transit vibration, and the effects of other agents will be more relevant concerns. Attempts to damage highly fragile stemware requires a combination of object vulnerability, sound intensity, and control that is not usual in commonly encountered situations. Based on the forgoing material detailing the forces imposed on fragile fresco structures from sound and other sources, ordinary handling would be a much greater concern for fragile items.
Indirect Force Effects
Vibration-induced Movement
One of the most important vibration effects to be aware of is simply the by-product of the movement it can cause. Vibration may originate from a variety of sources; two of the more important ones are earthquakes and vibration during transport. Note that shock may also cause vibratory motion; for example, ringing a bell. Some examples of configurations that may be vulnerable to the effects of vibration-induced movement are listed below:
- Unrestrained objects on a shelf that may move, fall, overturn, or collide with each other.
- Objects with loose components that collide with each other, e.g. impact of a heavy canvas against stretcher bars.
- Projections, assemblies, or other object components with existing damage that may concentrate the stress of any applied vibration.
- An object with fragile surface details that moves on a loosely fitted mount or that rubs against its cushioning during shipment (see Vignette 1.).
- Loose packages on a high stack that can fall inside a transport vehicle.
- Loose cargo on a transit vehicle that can bounce repetitively during transport (see Vignettes 2 and 3).
- Multiple objects inside a package, which are not properly separated, that collide with each other (note that this also contributed to the damage in Vignette 3).
Sources and Intensities of Force
For risk assessment purposes, it can be convenient to group sources of physical force in categories based on incidence and intensity. Four typical force categories are described below. The sources of force in each category appear in approximately decreasing order of severity.
Catastrophic forces (Low Incidence, High Intensity)
These forces have a low rate of occurrence, but they can inflict large-scale damage to large numbers of items if and when they do occur.
- Earthquake-induced ground movement is a source of potentially catastrophic damage in zones of moderate-to-high seismic activity. Note that a substantial portion of earthquake damage results from simple movement (as discussed above) as opposed to forces imposed by an earthquake's motions.
- War and vandalism may cause disastrous damage to structures, large numbers of objects, or individual works of art, especially popular or symbolic objects.
- Shipment disasters involving major vehicle accidents, e.g. overturning or a rear impact can cause severe damage to any object in transit.
- Extreme handling hazards such as accidents, intentional mishandling, and negligence that are not anticipated by typical prediction methods (e.g. probable drop height).
- Roof collapse due to snow or water accumulation, which can increase loads beyond structural design limits.
- Floor collapse under conditions of excessive load or load concentration (e.g. when moving or installing heavy objects).
Working forces (High Incidence, Moderate-to-High Intensity)
Forces encountered in daily activities with moderate-to-high magnitudes and incidence rates. The force magnitudes are usually predictable. They may affect one, several, or many items at a time.
- Handling. Manipulation of objects by manual or mechanical means, during movement, photo shoots, installation, crating, and uncrating.
- Transit (in-house). Impact, drops, and load concentrations when moving objects from one location to another inside a museum.
- Shipment. Moving packaged items from one venue to another, which includes handling (loading, unloading, cargo transfers) and the vehicle transport phases of shipment.
- Gravitational loads. Temporary or permanent distortion of objects as a result of gravity. Crushing or breaking object parts, distortion, and/or compressing object mounts. Distortion and failure of improperly designed pedestals or platforms for large, heavy objects.
- Construction vibration. Caused by nearby blasting or construction operations.
- Excavation. Structures may be damaged if the structures settle or shift due to lack of support near excavated areas.
Cumulative Forces (High Incidence, Low Intensity)
Low-intensity forces with a high (based on collection use rates) or continuous (e.g. gravity) incidence that inflicts ongoing damage to one or many items in a collection.
- Handling. Wear and tear caused by handling objects over time, especially objects made of, or which incorporate, poorly adhered, weak, fragile materials.
- Shipping. Low-level forces such as vibration inside packaging systems that affect object parts or surfaces.
- Gravity. Improperly designed supports that concentrate static loads on objects or their parts leading to distortion or breakage after an extended period of time.
Low-level Forces (Variable Incidence, Low Intensity)
These forces may raise concerns and cause annoyance to building occupants, but their direct action may not pose significant risks to objects.
- Building vibration from internally generated sources such as occupant activities, mechanical equipment, and nearby road traffic.
- Construction vibration from controlled blasting, distant blasting operations, pile driving, reciprocating equipment, and other construction activities.
- Acoustic sources that include audible or sub-audible, air-borne vibration, loud music.
A more detailed look at some of the more important sources of force among those mentioned above follows in approximately decreasing order of severity.
Ground Motion due to Earthquakes
The magnitude of an earthquake is measured on a Richter scale. A Modified Mercalli index is used to relate earthquake intensity to the apparent effects of the ground motion (Table 1). While the acceleration levels are low, earthquakes may involve substantial ground displacement and, therefore, earthquake acceleration levels are not directly comparable to acceleration levels from other sources in terms of damage potential.
Modified Mercalli Intensity Scale | Description of Effects | Maximum Acceleration (g) | Richter Magnitude |
---|---|---|---|
I | Not felt except by persons under highly favourable circumstances | - | M2 to M2.5 |
II | Felt by persons at rest, on upper floors, or favourably placed | - | M2.5 to M3.1 |
III | Felt indoors; hanging objects swing; vibration like the passing of light trucks occurs; might not be recognized as an earthquake | 0.003 to 0.007 | M3.1 to M3.7 |
IV | Hanging objects swing; vibration like the passing of heavy trucks, or there is a sensation of a jolt like a heavy ball striking a wall; standing motor cars rock; windows, dishes, and doors rattle; glasses clink; crockery clashes in upper range of IV; wooden walls and frames crack | 0.007 to 0.015 | M3.7 to M4.3 |
V | Felt outdoors; direction estimated; sleeping persons awakened; liquids become disturbed, some spill; small unstable objects are displaced or upset; doors swing, close, and open; shutters and pictures move; pendulum clocks stop, start, change rate | 0.015 to 0.03 | M4.3 to M4.9 |
VI | Felt by all; many are frightened and run outdoors; persons walk unsteadily; windows, dishes, glassware breaks; knickknacks, books, etc. fall off shelves; pictures fall off walls; furniture moves or overturns; weak plaster and masonry D (consult notes at the bottom) crack; small bells (church, school) ring; trees and bushes shake | 0.03 to 0.09 | M4.9 to M5.5 |
VII | Difficult to stand; noticed by drivers of motor cars; hanging objects quiver; furniture breaks; damage occurs to masonry Dincluding cracks; weak chimneys break at roofline; plaster, loose bricks, stones, tiles, cornices fall; some cracks appear in masonry C; waves appear on ponds, water turbid with mud; small slides and cave-ins occur along sand or gravel banks; large bells ring | 0.07 to 0.22 | M5.5 to M6.1 |
VIII | Steering of motor cars affected; damage occurs to masonry C with partial collapse; some damage occurs to masonry B, but none to masonry A; stucco and stone wall fail; Twisting, fall of chimneys, factory stacks, monuments, towers, and elevated tanks occur; framehouses move on foundations, if not bolted down; loose panel walls are thrown out; changes occur in flow or temperature of springs and wells; cracks appear in the ground and on steep slopes | 0.15 to 0.3 | M6.1 to M6.7 |
IX | General panic; masonry D is destroyed; masonry C is heavily damaged, sometimes with complete collapse; masonry B is seriously damaged; general damage occurs to foundations; frame structures shift off of foundations if not bolted to them; frames crack; serious damage occurs to reservoirs; underground pipes break; conspicuous cracks appear in ground; sand and mud ejected in alleviated areas; earthquake fountains and sand craters occur | 0.3 to 0.7 | M6.7 to M7.3 |
X | Most masonry and frame structures are destroyed; serious damage occurs to dams, dikes, and embankments; large landslides occur; water is thrown on banks of canals, rivers, lakes, etc.; sand and mud shifted horizontally on beaches and flat land; rails slightly bent | 0.45 to 1.5 | M7.3 to M7.9 |
XI | Rails are greatly bent; underground pipelines are completely out of service | 0.5 to 3 | M7.9 to M8.5 |
XII | Damage nearly total; large rock masses displaced; lines of sight and level are distorted; objects are thrown into the air | 0.5 to 7 | M8.5 to M9 |
|
Structural Considerations
Museums and museum storage facilities are often housed in buildings that were originally designed for other purposes. Two important structural issues to keep in mind are roof construction and floor-load capacity. Flat roofs without proper drainage may deflect and initiate a cycle that accumulates increasing amounts of water that can load the roof beyond its design limits. A flat roof adjacent to a peaked roof that sheds snow onto it may also be vulnerable to overloading. Floors for some museum applications must be strong enough to support the loads imposed by heavy objects or large collections. Note as well that lifting heavy items with equipment, such as gantry cranes, will concentrate loads onto relatively small areas of the floor. In some cases, it may be possible to shore up the floor from below to enable a heavy item to be lifted and safely moved along a pre-defined path. Wherever there is doubt, seek the services of a structural engineer. This is also a good precaution even if drawings or other specifications are available because these documents may not always describe the structure accurately or "as built."
Probable Drop Heights for Packages
The size and weight of any given item determines how it will be handled. Handling methods, in turn, can be used to predict hazard intensities during shipment. The packaging industry has assigned probable drop heights to package weights and sizes. Table 2 is a typical example. Probable drop height is a reasonable worst case scenario that is infrequent, and unlikely to be exceeded. Most of the drops that occur during shipment will be from much lower heights. Probable drop height estimates are derived from a long history of observations and experience in industry. Other estimates have been obtained from studies involving shipping packages containing electronic recording instruments (Allen ).
Package Weight (kg) | Greatest Dimension (cm) | Probable Drop (cm) | Form of Drop | Type of Handling |
---|---|---|---|---|
10 | 120 | 100 | Any side or corner | One man throw |
10 – 20 | 90 | 90 | Any side or corner | One man carry |
20 – 45 | 120 | 60 | Any side or corner | Two men carry |
45 – 70 | 150 | 50 | Any side or corner | Two men carry |
70 – 90 | 150 | 45 | Any side or corner | Two men carry |
90 – 270 | 180 | 60 | Rotating, either end roll or tip | Mechanical |
270 – 1360 | Unlimited | 45 | Rotating, either end roll or tip | Mechanical |
1360 + | Unlimited | 30 | Rotating, either end roll or tip | Mechanical |
Handling
Routine handling and accidental drops can easily generate damaging impacts and shock levels. The accelerations that may be expected at various drop heights for different packaging treatments are summarized in Table 3. Many items considered highly fragile, such as unfired clay, will sustain shocks of up to 50 g. Some items that are thought to be highly fragile can sustain even greater shock levels.
Drop Height cm | Unpackaged Metal container g | Wooden Box g | Carton g | 25 mm Cushioning g | 50 mm Cushioning g |
---|---|---|---|---|---|
120 | 392 | 196 | 131 | 98 | 52 |
110 | 367 | 183 | 122 | 92 | 49 |
90 | 339 | 170 | 113 | 85 | 45 |
60 | 277 | 139 | 92 | 69 | 37 |
15 | 139 | 69 | 46 | 35 | 18 |
5 | 80 | 40 | 27 | 20 | 11 |
Shock and vibration in transport vehicles
If packages are properly tied to the transit vehicle, the shocks generated by vehicle movement are roughly equal to a 15 cm drop. If packages are not tied to the transit vehicle, they may bounce repeatedly resulting in high-impact loads on the packages (see Vignette 3). Another cause of high impact to unrestrained cargo is simply the result of falling off a high stack due to cargo movement during transport. With proper restraint, the highest shock level that can be expected in transit vehicles occurs during the coupling of rail cars and from slack in the rail car couplings.
Vehicle vibration has been studied in detail by military and commercial investigators because all cargo on a transport vehicle will be subjected to vibration (the probability of vibration exposure is 100%) and, unlike handling hazards, vehicle vibration cannot be observed visually. A vibration summary for common modes of transport from Ostrem and Godshall appears in Figure 9. Please note: this information derives from laboratory testing of objects and packaging systems; however, the reference contains information of practical interest to the reader.
Vibration magnitude in most well-maintained transport vehicles is low and is generally regarded to be below the damage threshold of most commercial products in the absence of resonance effects. Among common carriers, truck transport generates the highest vibration magnitude and, therefore, has the greater damage potential. Vibration levels in air-ride trucks will be lower than in trucks with conventional suspensions. Because trucks are an integral part of almost all transport scenarios, data on their vibration environments has been used in experiments with models or art objects, such as canvas paintings. Overall, the secondary effects of vibration appear to be one of the most important issues during transport. (Consult the "Control Strategies" section for advice on how to control vibration effectively.)
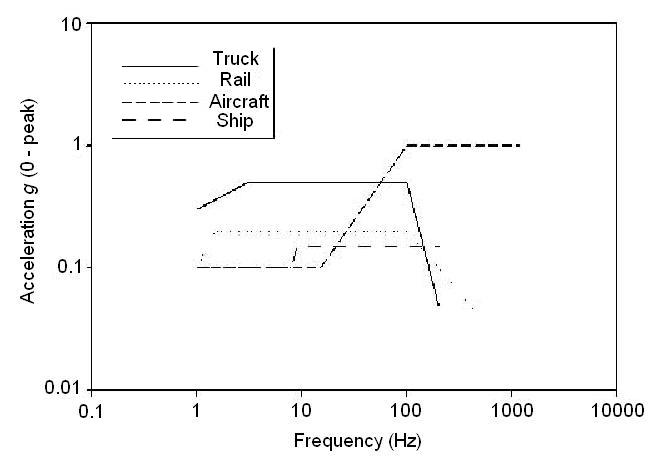
Gravity
Gravity imposes a force on objects in direct proportion to their mass. This results in a familiar quantity we know as weight (weight = mass × acceleration). Gravitational loads on objects or support materials may be concentrated by geometry.
Distortion of and strain on objects or their parts due to inadequate support may occur quickly, or over longer periods. Lifting large items will concentrate the gravitational loads at the lift points.
Construction Vibration
Investigations have been undertaken to quantify the vibration levels that damage buildings. Construction vibration literature indicates that most construction activity does not generate enough energy to cause structural damage in buildings and, as a result, the focus of almost all the damage investigations has been on blasting operations.
Table 4 contains data on vibration levels that cause three categories of building damage. The data was obtained from investigations involving new and old structures (and their substantial variations in condition, site details, and pre-existing strains). The same source provides data on strain levels (expressed as equivalent particle velocities) induced by building occupant activities, environmental changes, and blasting. This shows some interesting results — specifically that some of the highest strain levels recorded were the result of building occupant activities and daily changes in temperature and humidity. Some additional points concerning blast-related cracking in buildings are outlined below (Dowding ):
- Cracks are caused by a variety of construction defects.
- Buildings will contain many cracks, which the occupants may not be aware of, that will increase in size and number each year in the absence of vibration.
- Most of these cracks are cosmetic and are not structurally harmful. (Author's note: Wherever there is concern about the appearance of cracking and there is any doubt about its significance, an expert investigation should be carried out.)
- Slamming doors and passing traffic may vibrate buildings more than noisy blasts or pile driving.
- Humans are more sensitive to vibration than structures.
Effect | Particle Velocity (mm/s) | Description of effect |
---|---|---|
Threshold | 76 | Opening of old cracks and formation of new plaster cracks; dislodging of loose objects (e.g. loose bricks in chimneys) |
Minor | 114 | Superficial, not affecting the strength of the structures (e.g. broken windows, loosened, or falling plaster); hairline cracks in masonry |
Major | 203 | Serious weakening of the structure (e.g. large cracks or shifting of foundations or bearing walls, settlement resulting in distortion or weakening of the superstructure) |
Wherever there is concern about the effects of construction activities, pre-construction crack surveys can help establish a clear connection between any damage that is observed and the forces generated by construction activity. Where naturally occurring crack development is ongoing in the absence of vibration, changes in the rate of crack development may be informative. Detailed information on crack observation and documentation methods is available (Dowding ).
Fragility of Objects
Having information on object fragility is an essential part of risk assessment. Hazards can then be limited to force levels that are at an acceptable margin below levels that cause damage. Beyond this, there is little advantage in trying to eliminate shipping forces entirely and trying to do is rarely feasible in terms of cost. Because art differs widely in materials, construction, and condition, making accurate fragility assessments is a harder task than it is for commercial products. Investigations into the shock and vibration sensitivity of canvas paintings, plaster, clay pottery, and some glass objects have been carried out. A useful base of knowledge on this subject is emerging. Despite the lack of accurate fragility data for many object types, it is still possible to make reasonable fragility estimates and mitigate hazards in a way that is satisfactory for a chosen method of shipment.
Shock Fragility Estimation by Comparison to Commercial Categories
The use of commercial fragility information has been helpful for making inferred estimates for some museum objects. Six fragility categories for commercial items with museum objects juxtaposed are illustrated in Table 5. The museum examples shown are the outcome of experience-based estimates and experimental investigations. These observations have shown that many museum objects will be safe inside packages that are designed to limit shocks for worst case drop hazards to about 50 g or less.
Category Description | Commercial Product Examples | Museum Object Examples | Fragility range (g) |
---|---|---|---|
Extremely Fragile | Missile guidance systems, precision-aligned test instruments | Plaster sculpture; 120 kg, hollow plaster test model damaged at 20 g. Other sculpture scenarios may be more fragile | 15 to 25 |
Very Delicate | Mechanically shock-mounted instruments and electronic equipment | Unfired clay (greenware) and fragile glassware | 25 to 40 |
Delicate | Aircraft accessories, electric typewriters, cash registers, and other electrically powered office equipment | Unfired clay, low-fired clay, plaster, stucco, glassware, ceramics | 40 to 60 |
Moderately Delicate | Television receivers, aircraft accessories | Very weak, damaged, and flaking paint on a 60 x 60 cm painting is dislodged by a corner or edge impact | 60 to 85 |
Moderately Rugged | Laundry equipment, refrigerators, appliances | Uncracked, brittle 60 x 80 cm canvas painting with brittle (low glue content) gesso develops cracks during corner drop | 85 to 115 |
Rugged | Machinery | 60 x 80 cm canvas painting (topple, edge drops when equipped with a protective backing board). Small partially adhered canvas paint flakes do not delaminate; tolerated by small boxed collections of pinned insects | 115 and up |
Object Characteristics and Sensitivity to Forces
The following parameters influence the sensitivity of any given object to force. Knowing this can help anticipate the susceptibility of unusually fragile items or items for which fragility data is not available.
Mass
As an object's mass increases, so does the force that acts on it for any given level of acceleration (Newton's Second Law F = ma, where: F = Force, m = object mass, a = acceleration). This explains why small, light, paint flakes may not fly off of a canvas at low to moderate levels of shock or vibration because considerable acceleration is needed to overcome even a relatively weak adhesive force that holds a lightweight particle in place.
Material Weakness
Material weakness makes an object highly sensitive to direct handling. Note that material weakness does not always result in a high sensitivity to shock or vibration. Some items, such as pastel paintings, are almost impossible to touch without damaging them, yet the very low mass particles on some older (as opposed to some recently made) works may not be easily dislodged by shock. The shock sensitivity of an object made of weak material will, however, increase with increasing mass. A good example is unfired clay (greenware), which is highly sensitive to both handling forces and shock.
Geometry
As the complexity of an object's geometry increases, so does its susceptibility to force. Projections often create sites where forces (and stress) can multiply in object materials.
Flexibility
Flexibility in objects can be considered a structural weakness. Wherever flexibility exists, there is a tendency to vibrate. This makes the object susceptible to the force multiplying effects of resonance or to damage when moving parts collide. The control of obvious vibration tendencies by gentle but firm restraint is an important part of avoiding shipping damage to fragile objects. Good mount-making practice will also help avoid any vibration tendencies (Figure 10).
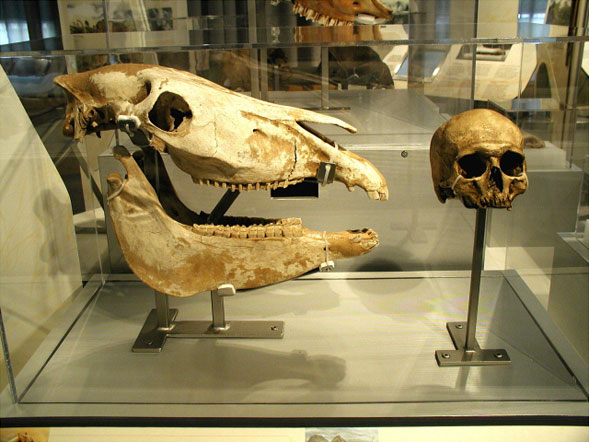
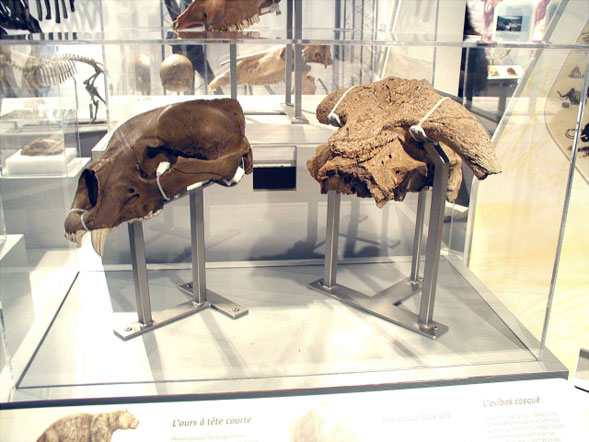
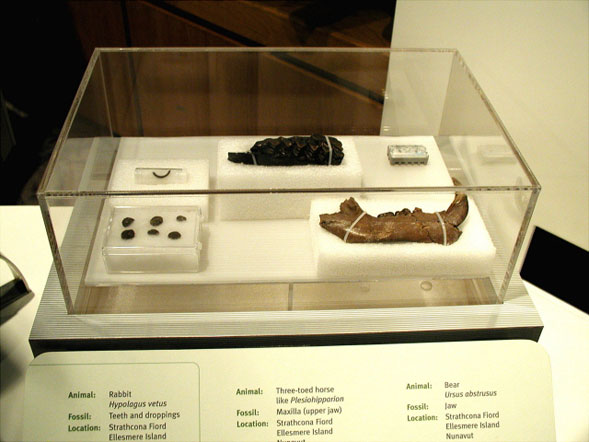
Vibration Susceptibility
Vibration fragility ratings for commercial products specify damage thresholds in terms of frequency and amplitude. Another method of vibration susceptibility used in the packaging industry involves determining the first few resonant frequencies of an object in the transport frequency range of 1 to 200 Hz. Vibration tendencies in this range may be of concern due to possible resonance effects that can amplify low-level transit vibration to damaging levels. The first few frequencies of the object are usually of greatest interest because lower frequencies involve the largest displacements and strains.
Examples of Object Susceptibility to Handling, Shock, and Vibration
The following examples indicate the force susceptibility of some different object types. Because materials, compositions, and configurations of actual objects vary widely, these are considered general examples.
High sensitivity to manual handling due to material weakness
Objects made from lightweight, weak materials in any mass range are susceptible to forces due to gravity (point loads, improper support) and direct handling:
- Pastel paintings (handling, abrasion).
- Paintings with fragile surfaces (handling abrasion).
Moderate handling sensitivity, low shock, and vibration sensitivity
Strong, low-mass materials and assemblies:
- Small pinned insect display
High handling sensitivity with shock susceptibility
Where material weakness exists or if moderately strong materials are stressed by mass, geometric complexity or a combination of both:
- Large pinned insect displays
- Unfired clay, terracotta, plaster, and stucco (handling, abrasion, shock).
- Some ethnographic materials (handling, abrasion, shock, and vibration).
- Paintings with cracked fragile and reasonably thick (massive) paint layers.
- Some contemporary artwork, kinetic artwork.
High sensitivity to shock and vibration
Mass and geometric complexity combine to make these objects fragile:
- Assembled skeletons, composite objects.
- Clocks with unrestrained mechanical components.
- Contemporary art (e.g. fragile glass Terella, see Figure 16) with inaccessible, internal components that vibrate at low frequency).
- Canvas paintings (shock resulting in stretcher bar deformation, low-frequency vibration that may lead to secondary effects such as abrasion or collision).
High sensitivity to mechanical lifting and load concentration, high shock (primarily), and vibration sensitivity
This result is due to mass, geometry, and material properties: mass augments the effects of forces on compact forms of moderate to strong materials. Geometry and notch sensitivity in brittle materials further increases the sensitivity of strong materials to direct force, shock, and vibration:
- Large paintings (mass-driven stretcher bar deformation, collision of canvas with stretcher bars).
- Sculpture (high mass, brittle, notch-sensitive materials, projections).
Control Strategies
An effective preservation strategy involves setting up control measures to counteract the action of potentially damaging forces. The general strategies for basic, medium, and high-level control strategies are summarized below. They also incorporate priority issues for force control, which are also listed below in approximately decreasing order:
- Earthquake (stabilization of buildings, hardware, and objects in high-risk areas). See Vignette 4.
- Structural details (floors, roofs, artifact supports, hardware).
- Handling (crating, uncrating, moving in-house, isolation from public).
- Shipment (shipping hazards).
- Long-term wear (cumulative forces in storage or from repeated handling).
Control of damaging force levels is possible by a variety of individual features at three different levels of implementation: buildings, hardware, and procedures. Building level controls are the most expensive to achieve. Large institutions will incorporate many of their control features here. Effective control is also possible at lower cost at the hardware and procedural levels. For any level of implementation, individual features will enable a sequence of control stages to Avoid, Block, Detect, and Respond to the action of forces agent. Each stage in the control sequence comes into play whenever a preceding one is either not feasible, not possible, or fails. This establishes an effective line of defense against physical forces or other agents of deterioration. A general summary of control features by level of implementation appears below. Table 6 lists features by scale under the various four control stage headings.
1. Basic (Control of major risks)
Buildings
- Earthquake-resistant buildings in zones of seismic risk.
- Consider all non-structural hazards (including monumental items such as sculpture) in seismic zones and secure as necessary.
- Ensure seismic stability in the design of pedestals, hangers, and mounts for large objects in seismic risk zones.
- Good, structurally sound buildings with adequate floor strength. Ensure that the use of existing buildings is appropriate for museum application.
- Attention to basic building design features (halls, doorways) to promote good access.
- Avoid flat roofs.
- Re-locate vibration-sensitive equipment to ground floors, near load-bearing walls, or near support columns on upper floors to avoid vibration problems.
Hardware
- Rigid shelving and stabilization of all objects in seismic areas.
- Provide moving equipment (e.g. dollies, carts, lifts, slings) for prompt evacuation of objects where necessary.
- Basic isolation between the public and objects on display (cases, barriers).
- Specialized packaging for highly fragile or vulnerable items.
- Select appropriate storage surfaces and mounts for specific items.
- Use specially designed (high mass) tables or vibration isolators for sensitive equipment.
Procedures
- Use specialized art handler's carriers for highly valuable or fragile items, appropriately crated.
- Primary packaging on a basic level to protect selected fragile items from puncture, dent, scratches, abrasion, and minor impact. (Primary protection refers to a basic packaging treatment that enables a fragile item to be handled easily. Protecting the item during shipment normally requires additional packaging.)
- Identify and protect highly susceptible items from routine handling while in storage, during display, and in transit.
- Secure all objects or packages in vehicles during transport.
- Provide staff training on handling fragile museum objects.
- Provide staff training in machine operation and on handling objects (rigging) up to 2,300 kg or hire experienced handlers (riggers) for loads greater than 2,300 kg.
- Acquire training and expertise on packaging requirements for vulnerable items.
- Provide security staff in exhibition areas.
- Ensure that object surfaces are clean before packing to avoid abrasion or forcing dirt into object surfaces.
2. Medium (Control for moderate-to-high intensity forces)
Buildings
- Provide specialized loading bays and handling equipment such as smooth lifts, load levelers, smooth soft floor, and wall finishes.
- Provide impact-absorbing door perimeters.
Hardware
- Provide gantry cranes for moving or re-orienting heavy objects.
- Ensure seismic stabilization of small-to-medium-size objects.
- Transit framework and modular systems for transport and handling large objects that travel. This may take the form of incorporated features that enable large, heavy items to be easily moved during a multi-venue exhibition.
- Primary packaging with the basic benefits that it provides (as discussed above).
- Basic packaging — good crates with adequate (typically at least 50 mm) thickness of cushioning material.
- Well-designed mounts for storage, transport, and display.
Procedures
- Staff training in object susceptibility, object handling, mountmaking, and packaging.
- High-level isolation of fragile items from the public in display areas (effective barriers, cases, alarms).
3. High (Control of All Perceived Risks of This Agent)
Requires all of the above described control strategies plus implementation of collection-specific needs dictated by a comprehensive collection survey. Rank order and implementation of relevant building hardware and procedural features for collection.
Preventive Conservation Framework
There are many ways to implement an effective control strategy against forces. Table 6 is an excerpt from the Framework for Preservation of Museum Collections, which identifies nine additional agents of deterioration and the control features for each agent. Many control features for forces are found in the Block control stage.
Hardware | Buildings | Transit | Procedures |
---|---|---|---|
AVOID
|
AVOID
|
AVOID
|
AVOID
|
BLOCK
|
BLOCK
|
BLOCK
|
BLOCK
|
DETECT
|
DETECT
|
DETECT
|
DETECT
|
RESPOND
|
RESPOND
|
RESPOND
|
RESPOND
|
Protecting Fragile Objects during Shipment
After seismic forces and forces affecting structures, in-house handling and shipment are the usual highest commonly encountered level of force magnitudes that museum objects encounter. Staff training, planning, building, and hardware features all contribute to reducing the risk of damage from these sources. A general overview of important issues related to handling and shipment follows.
Avoid fundamental deficiencies
Serious damage to packaged items can result from fundamental oversights. Simple matters such as a container's durability, accounting for how packaged objects may move and interact with each other, and securing packages to the transport vehicle can make a big difference.
Cargo restraint
Unrestrained, stacked cargo in vehicles can fall and experience higher than anticipated drops. In one example of severe damage, unrestrained cargo simply slid off a truck (see Vignette 2).
Unrestrained cargo can also bounce, shift, and collide repetitively resulting in high-impact forces (see Vignette 3). Content movement inside unrestrained packages may also result in damage (another cause of the damage described in Vignette 3).
Avoid unnecessary forces
Transit forces are impossible to avoid, but carrier choices can make a big difference in the forces that act on packages during shipment. One shipping study comparing an art handler shipment with common carriers did not record a single shock event for the art handler shipment (despite a very low recorder activation threshold of 5 g). When shipping numerous items, high-quality transport and handling can save money, by reducing packing and crating requirements (consult Figure 10). Crate design features can also help reduce handling hazards. Such features include handles at appropriate positions (height) to help minimize the height from which a package may be accidentally dropped; and clearance provided at the base of cases to enable the use of pallet jacks (helps avoid unnecessary strain and possible deformation of large containers and strain on their contents. Lightweight packages, that are transportable inside an institution, can also protect objects until they reach their ultimate exhibition location.
Assess hazards for your distribution network
Each distribution network carries its own profile of risk. It is reasonable to expect fewer and less severe impacts during art handler shipments. Investigations using packages containing data loggers have been able to show the difference. Common carrier shipments will usually call for greater package durability and better cushioning than do art handler shipments. Tabulated data from commercial sources on probable drop heights for different package weights or size are available (consult Table 2). The tabulated values indicate the maximum probable drops that might be expected; however, note that these are infrequent events. Less than 5% of drops will ever exceed these values (according to the Institute of Packaging Professionals [IoPP]).
Decrease the objects susceptibility to force
Commercial products are usually redesigned if they fail to meet basic durability requirements in order to reduce packaging costs. Identifying and addressing potential weaknesses in the object will protect it outside the shipping crate. Examples might include disassembling fragile vibration-prone components, and restraining vibration-prone items, the latter being common practice in commercial shipments. Treating artwork to increase its durability and adding features such as protective backings for paintings that "stiffen" the canvas painting structure (Figure 11 and 12) will decrease packaging performance requirements. A stretcher lining treatment is a lightweight alternative for larger paintings (Figure 12).
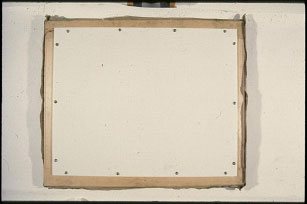
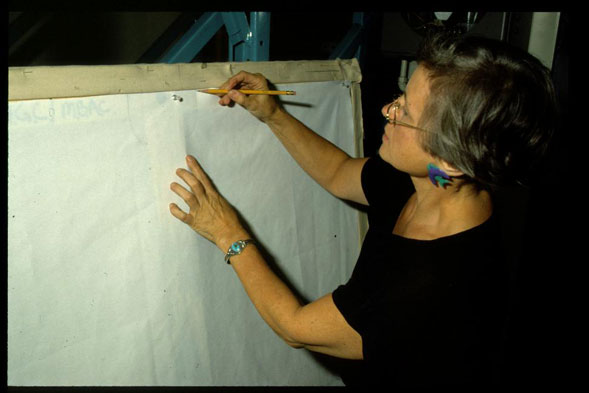
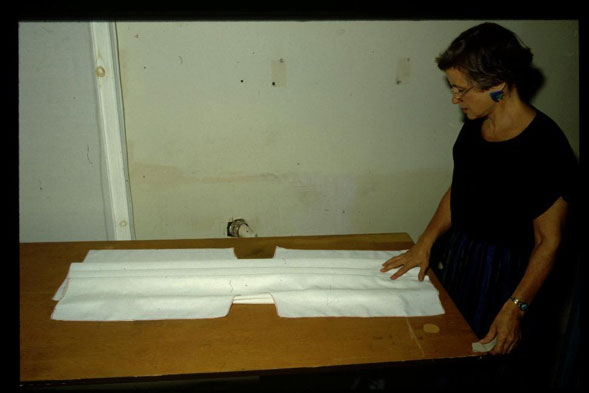
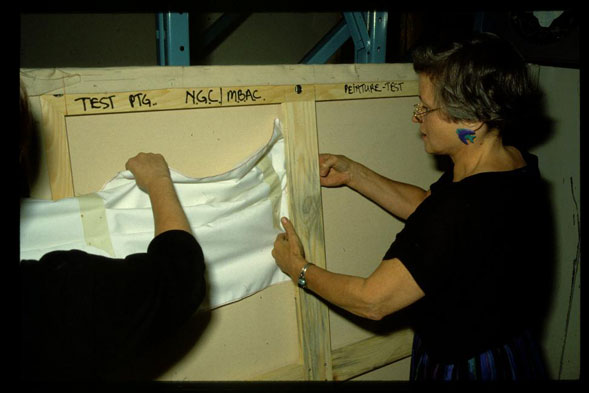
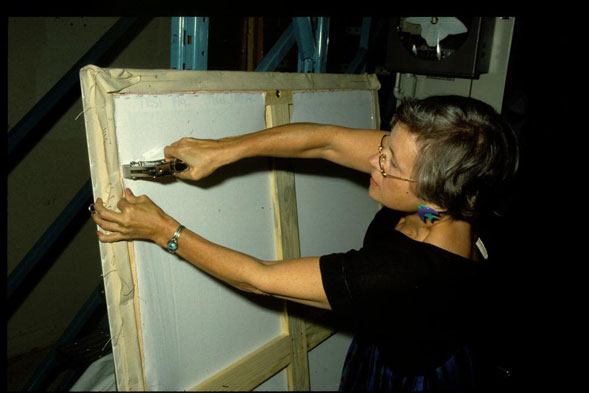
Backing board | Corner Drop (g) | Topple (g) | Edge Drop (g) | Flat Drop (g) |
---|---|---|---|---|
No | 65 | 80 | 100 | 100 |
Yes | 190 | Topple from 90° without damage | 100 (estimated) | 200 |
Condition | Resonant Frequency (Hz) | Displacement (centre of canvas for a constant vibration amplitude) (mm) |
---|---|---|
Painting | 5 | 100 |
Masonite backing board | 16 | 18 |
Fluted plastic (with centre attachment) | 18 | 15 |
Fabric lining (illustrated above) | 20 | 3 |
Aluminum honeycomb | 24 | 8 |
Provide primary protection
Fragile objects benefit from supports that enable them to be handled and transported easily (Figure 13). Lightweight mounts and enclosures protect against direct handling forces and direct application of force that may lead to scratches, dents, punctures, and abrasion. Fabricating custom supports can be time consuming, but the supports offer long-term protection if made from archival-quality materials. In some situations, the primary packaging may be used alone for local transport, with careful handling, or packed and cushioned as larger units in larger containers for longer travel distances or multiple venue exhibitions. Finally, a primary packaging system can help transfer the movement away from fragile object surfaces (e.g. a firm-fitting mount that is supported on flexible cushions should avoid movement along the object–mount interface).
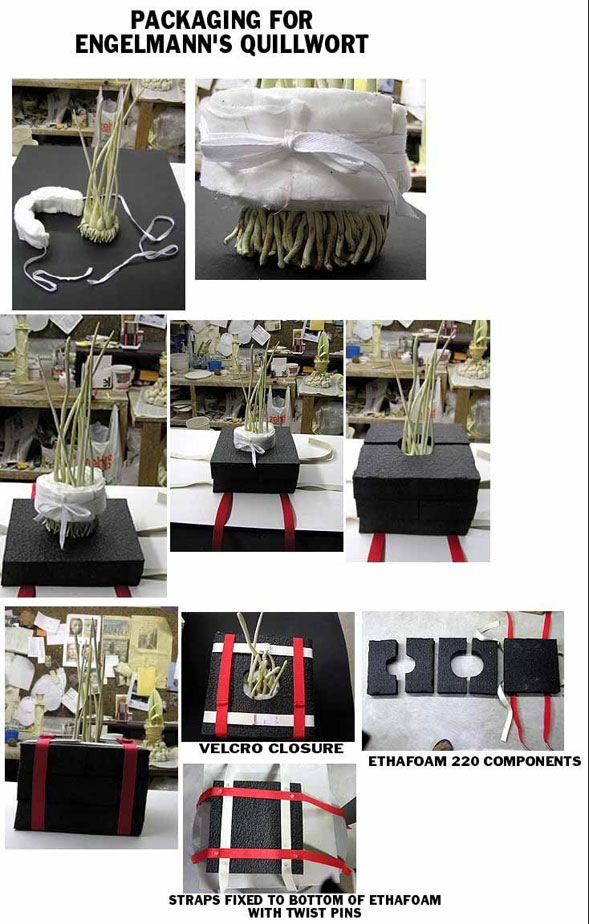
Provide basic cushioning
When a cushioned object is dropped, it will gradually sink into the cushioning material on impact. The object decelerates gradually, which limits the forces on the object to levels it can sustain without damage. A successful outcome requires an appropriate material choice to enable the object to deflect into the cushioning material without bottoming out and striking the inside of the packing case. A 50-mm thickness of almost any cushioning material applied to all sides of an object (weighing 75 kg or less) can go a long way to ensuring its safe shipment. Bubble pack is the least labour-intensive material to use for small lightweight items. Foams, such as polyurethane and polyethylene, also have excellent cushioning properties. Loose fill materials may be used, but be aware that objects cushioned in this material may travel toward the interior surfaces of the container and there may not be any cushion material present if and when it is needed (Figure 14).
Figure 14. Reproduction glasses made of soda lime glass. Individual boxes containing loosely bubble-wrapped glasses moved in the peanut packaging material and collided, resulting in damage to the pieces.
Use Cushion Design Methods and Data
Industry provides detailed performance data for most cushioning materials and a step-by-step procedure for designing protective cushioning. The falling clay pot demonstration illustrates how well cushioning materials can perform if properly selected (Figure 15). Cushioning data is published by material manufacturers and other sources in the form of dynamic cushioning curves, which are easy to interpret after a bit of practice. User-friendly design tools based on these curves have been developed to simplify the design process while eliminating the need to interpret graphical data, compare different materials, and perform repetitive calculations.
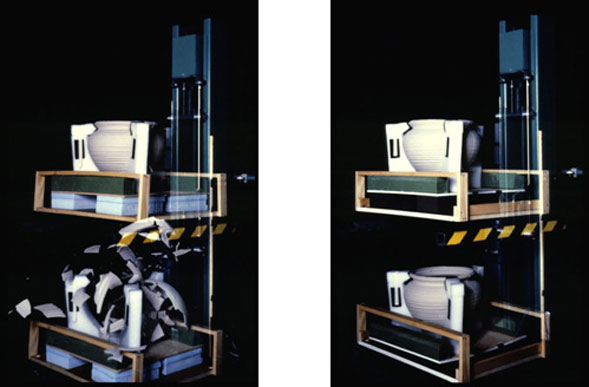
a) The polyethylene foam support and blue polystyrene pads (cushioning) provide some protection, but not enough for this highly fragile item.
b) Cushion performance data indicates that a set of ether type — polyurethane (24 kg/m3 density) pads cut to the same size and thickness as the four polystyrene pads shown — will limit shock to less than 40 g. This is enough to protect the pot against repeated 75 cm drops (note the deflection of the pads on impact). Cushioning works by giving the pot more time to come to a stop on impact. This limits the impact forces to a level that an object can sustain without damage.
Consider dynamics of the packaging system
All package components work together to protect fragile items. The shipping container is the first line of defense against punctures, dents, and abrasion. Treatment of the object's surface and how the object is supported/restrained on a cushioning material inside a package also plays an important role in the overall effectiveness of the packaging system. A key requirement for cushioning against shock is to ensure that that the object can sink into the cushion material on impact. The cushioned object must be able to move freely while avoiding excessive looseness. Care should be taken to ensure that projections are not strained as the object moves or deflects into the cushioning material and that fragile surface finishes will not be abraded. Object mounts or fixtures can transfer the movement away from fragile surfaces and projections.
Vibration Control Measures
There are three ways of controlling vibration hazards during shipment:
- Source reduction such as choosing air-ride vehicles and well-maintained fleets.
- Vibration response of the object altered, e.g. by providing gentle restraint of vibration-prone assemblies and disassembly.
- Vibration isolation, e.g. by designing cushioning systems to isolate resonant frequencies of objects.
The choice of air-ride vehicles over those with conventional suspension systems will reduce the amount of transport shock and vibration that packages will receive. The painting treatments in Figure 11 and Figure 12 are a form of restraint. Direct restraint of vibration-prone items may be possible for other objects. Disassembly may also be an option in some cases. A cushioning system designed for shock isolation can also provide vibration isolation. A key requirement for achieving this outcome is that the cushioning system has more flexibility than the cushioned item it protects. See Figure 16 for an interesting example involving an inaccessible, flexible component.
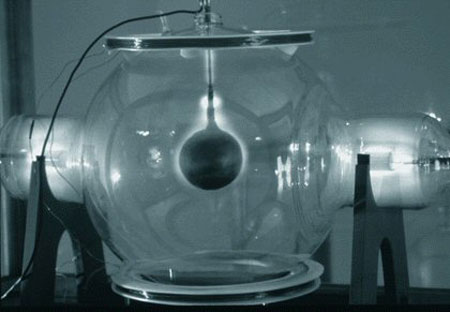
Double crating
Double crating can simplify packaging for items with irregular shapes or fragile surfaces (Figure 17). The procedure involves fitting the object firmly onto a transit mount or inner crate that can gently restrain it in all directions and that has simple flat exterior surfaces. Protective cushions can then be easily applied to the inner box (or assembly), which then fits into the shipping crate. Many package designs for highly fragile items have been designed this way and all have performed very well.
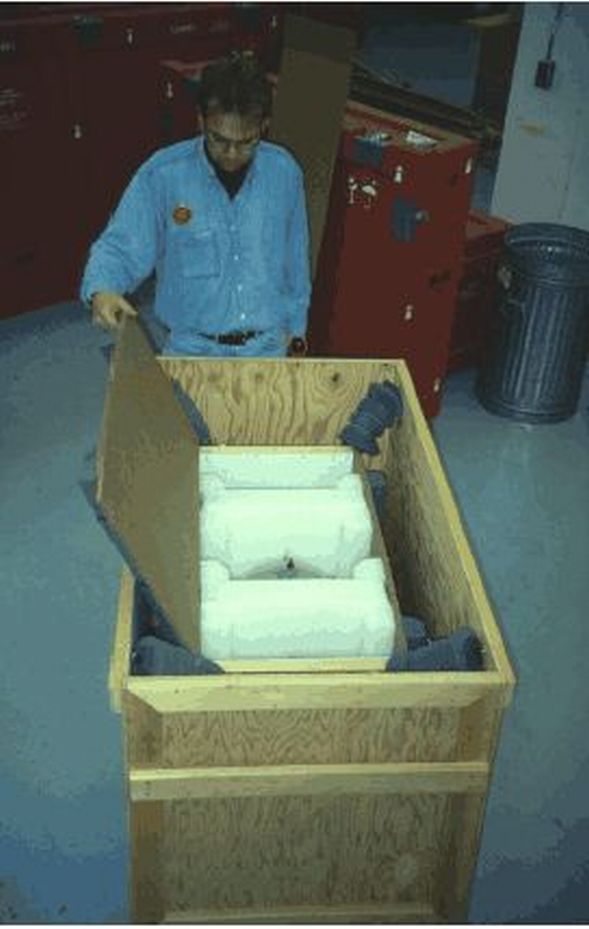
Evaluate overall package performance
A packaging system should be easy to use and should also perform as expected. Packages for multiple venue exhibitions benefit from simple designs with clearly marked removable components that are easy for third parties to uncrate and repack. The package should be easy to open and close without applying force to the contents and without binding the cushioned object inside and restricting its movement. A basic evaluation of crate performance is possible without elaborate instrumentation by simply dropping a crate with simulated contents onto a hard floor using a safe release mechanism (ASTM 2001). Monitors can also evaluate the effectiveness of a cushioning system using simulated (by weight and size) package contents. These monitors may range from inexpensive resettable shock indicators costing a few dollars each to electronic data loggers, which can record shock pulse information and display the results through computer software.
Vignettes
Vignette 1. Venus Blue (Fragile surface damaged in transit)
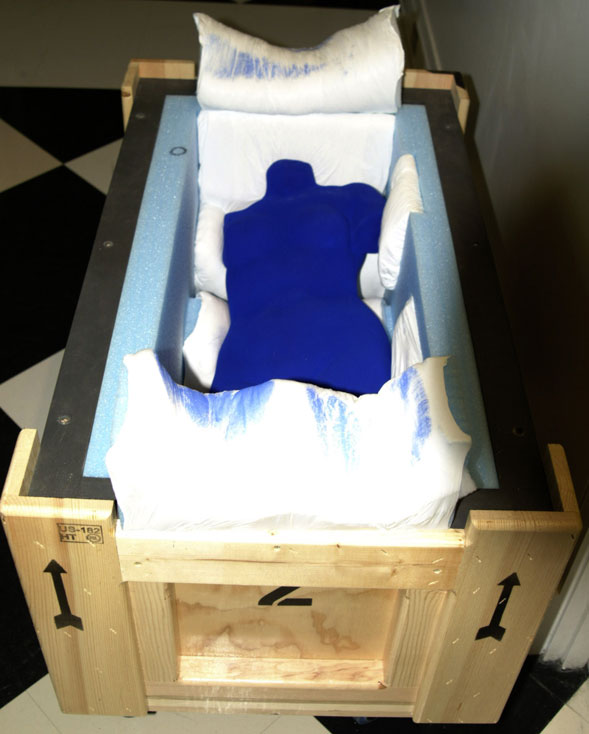
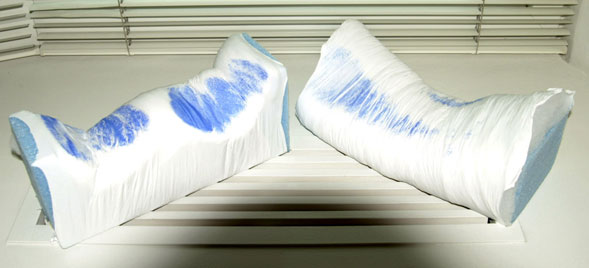
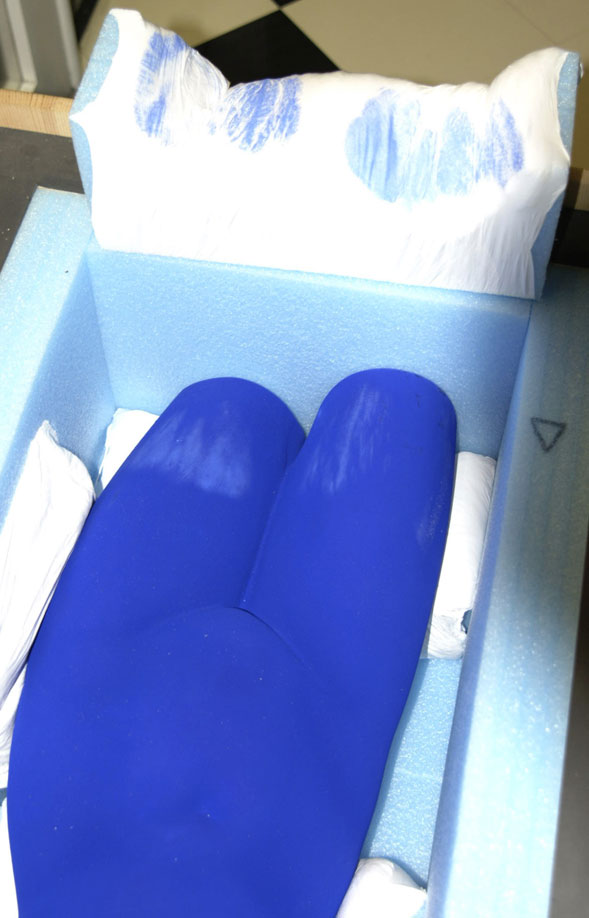
A hollow plaster figure with a powdery paint surface was packed inside a very well-made shipping container that was transported by an art handler. While this treatment is enough to guarantee success in most cases, the object arrived at the exhibition with substantial surface impressions and could not be displayed. The cushioning method that was used involved floating the object directly on polyethylene foam pads that were covered with a Teflon sheet, which was used to minimize abrasion. Movement between the object and the Teflon sheet and load concentration caused impressions in the pigment. This might have been avoided by fitting the sculpture into a negative mount and floating this combination on pads to eliminate any movement of the object and minimize the load on its fragile surface. An approach such as this was successful for protecting hollow plaster sculptures with fragile surfaces (Marcon ). Fortunately, in this case it was possible to eliminate the surface impressions on the Venus Blue with a conservation treatment.
Vignette 2. 7.5 Million-year-old Dinosaur Specimen Smashed in Transit
A transport truck backing down a inclined ramp at a museum had its back doors open. Inside the trailer was a dinosaur fossil that was recently restored at a cost of $250,000. The crate slid out of the truck when the brakes were suddenly applied. It fell onto the concrete ramp, smashing the fossil to pieces. The package, while suitable for most routine handling, did not provide adequate cushioning for a drop from tailgate height. Damage would have been avoided if the package had been securely tied to the transport vehicle or if the package had been designed for a worst case drop from tailgate height.
Vignette 3. Furniture Shipping Damage (Martha Sturdy, Vancouver, British Columbia)
A Vancouver-based furniture designer shipped acrylic resin samples to a New York trade show inside a very large shipping container. The substantial resin pieces were 50 mm thick and arrived at the destination in pieces. Subsequent investigation revealed that the large shipping container was not tied to the transit vehicle. There is evidence that it moved repeatedly during shipment. This movement, combined with impact between relatively heavy contents that were individually wrapped in thin layers of bubble pack, was the cause of damage. An interesting video documentary on this case is available from the Canadian Broadcasting Corporation (CBC).
Vignette 4. Seismic Resilience for Artwork and Antiquities
(written by Jay Lewis, Terra Firm Earthquake Preparedness)
Many of the world's museums and art galleries are located in large urban areas with the potential for strong earthquakes. The extensive collections of artwork and antiquities can be damaged or lost due to falling, collision with other objects, loss of environmental control systems, water damage, or fire. Most of this risk is controllable by using engineering-based, seismic risk-mitigation techniques.
Earthquake Forces
During an earthquake, ground motion may be horizontal in more than one direction as well as vertical. An art object, which is not attached to a building, through inertia, will attempt to stay where it is located as the building moves with the ground. This results in the artwork toppling, sliding, or falling with the resulting damage when it strikes the building or other objects. The seismic forces are also acting on the building, which can trigger falling ceiling systems and structural elements, broken water lines, and fire ignited by failing electrical and fuel systems.
Options for Seismic Risk Mitigation
There are a number of options for mitigating the impact of an earthquake on artwork and antiquities. These can be grouped into the six "Rs" of seismic risk mitigation in order of impact and cost.
Remove. If the object is not an essential display element, it can be removed from its location and stored away, traded, or sold.
Relocate. There may be safer places within the building for a particular object. Threats in a particular location might be due to another piece of artwork at risk of toppling or a large overhead water pipe. By relocating items within the facility, risk can often be reduced at little cost.
Replace. If there is a decision to be made between a tall and thin item balanced on a thin point and a low and broad item, the latter is the best seismic choice. This is not a choice the curator often has, but, in general, fragile items present more risk that durable ones. Substitution might be a solution, all other factors being equal.
Reinforce. Without affecting the artistic value of the work, some things can be reinforced to be more durable. This can allow the work to resist seismic forces without significant damage.
Reduce. By reducing the seismic forces acting on the artwork, the risk is reduced. This can be accomplished by damping and base isolation. With damping, the forces are partially lowered using energy-absorbing devices so that other mitigation measures are reduced or avoided. By isolating the base, the object is virtually disconnected from the building. In some cases, the entire building is isolated. This approach uses rubber or roller bearings to do the job. Relatively high cost can be an issue if choosing isolation.
Restrain. The last on the list is to restrain an object so that it moves with the building and cannot topple, slide, or fall. This can be done by confining or fastening the object. Confining may be preferable because it does not involve penetrating the work. On the other hand, restraint features can have significant visual impact depending on the design. Restraint has been associated with the conservator's profession for a very long time. Items such as conservation wax, tapes, and specialized display cases are commonly used. Other techniques and products are being introduced to the market on a regular basis.
Engineered Solutions
As the prices of artwork and antiquities rise and the liability associated with health and safety issues in public places increases, effective seismic risk mitigation becomes an important element in the curator's job. Because the procedures are complex and demonstrating due diligence is required, the conservator will increasingly consult with a structural engineer for standard and custom solutions and details. Each facility should conduct a preliminary seismic risk assessment of its collection using a commonly accepted procedure such as found in the Canadian Standards Association's (CSA) S832 "Seismic Risk Reduction of Operational and Functional Components (OFCs) of Buildings" (Canadian Standards Association ). The CSA also originated the six "Rs" approach to seismic risk mitigation paraphrased above. A prioritized list has been established for establishing a mitigation plan and developing annual budgets for the work. This process establishes a clear due diligence path and reduces risk quickly, at the least cost. Maintaining the mitigation systems, once installed, is an ongoing effort, which requires regular attention and funding.
When choosing a mitigation system, it is important to clearly identify the objectives of the work in advance. This includes identifying limitations on attachments to the item (such as drilling holes), aesthetic or visual quality impacts, and performance objectives (such as life safety, protection of the asset, or both). Having these parameters clearly identified ensures that the design professional pursues solutions that are appropriate for the circumstances. No museum or gallery in an earthquake zone should be without a comprehensive seismic risk mitigation program.
References
- Agbabian, M.S., et al. Evaluation of Seismic Mitigation Measures for Art Objects. GCI Scientific Program Report. Los Angeles, CA: J. Paul Getty Institute, .
- American Society for Testing and Materials. Annual Book of ASTM Standards, Volume 15.09. Philadelphia, PA: ASTM, . (Detailed drawings for a safe release apparatus are available from ASTM, 1916 Race Street, Philadelphia, PA 19103. Request Adjunct Nº 12-409970-00.)
- Booth, P. "Stretcher Design: Problems and Solutions." The Conservator 13 (), pp. 31–40.
- Canadian Broadcasting Corporation (CBC). "Tempest in a Packing Crate." Aired on on "CBC Venture." Toronto, ON: CBC Visual Resources Centre, .
- Canadian Standards Association. Seismic Risk Reduction of Operational and Functional Components (OFCs) of Buildings. CSA S832. . Canadian Standards Association.
- Castellini P., et al. "Non Invasive Measurements of Damage of Frescoe Paintings and Icons by Laser Scanning Vibrometer: Experimental Results on Artificial Samples Using Different Types of Structural Exciters." Ancona, Italy: Dipartemento di Meccanica, Universita di Ancona. Vol. 4, Nº 12, 6th World Conference on NDT and Microanalysis in Diagnostics and Conservation of Cultural and Environmental Heritage, Rome, .
- Dowding, C. Construction Vibrations. . ISBN 0-99644313-1-9.
- Harris, C.M. Shock and Vibration Handbook. 3rd edition. New York, NY: McGraw-Hill Publishing Co., , p. 44-23, Figure 44-20.
- Marcon P., et al. "Packing and Transport of Hollow Plaster Sculpture." pp. 77–82 in ICOM Committee for Conservation 12th Triennial meeting Lyon, – : Preprints. Vol. 1. London, UK: James & James, .
- Michalski, S. "Paintings – Their Response to Temperature, Relative Humidity, Shock and Vibration." pp. 223–248 in Art in Transit, Studies in the Transport of Paintings (edited by M.F. Mecklenburg). Washington, DC: National Gallery of Art, .
- Michalski S., and P. Marcon. "Mechanical Risks to Large Paintings such as Guernica during Transit." pp. 87–98 in El Guernica y los problemas eticos y technicos de la manipulation de obras de arte. Santander, Spain: Fundacion Marcelino Botin, .
- Northwood, T.D. "Isolation of Buildings from Ground Motion." pp. 87–102 in Proceedings of the Isolation of Mechanical Vibration, Impact and Noise Colloquium. Presented at the American Society of Mechanical Engineers (ASME) Design and Engineering Conference, Cincinnati, OH. New York, NY: ASME, United Engineering Centre, .
- Packing and Crating Information Network. Technical Drawing Handbook of Packing and Crating Methods. Packing and Crating Information Network.
- Stagg, M.S., et al. Effects of Repeated Blasting on a Wood Frame House. Report of Investigations 8896. Washington, DC: U.S. Bureau of Mines, .
- U.S. Department of Defense. Military Standardization Handbook, Package Cushioning Design. MIL-HDBK-304B. Washington, DC: U.S. Department of Defense, .
- Ungar, E.E. Vibration Criteria for Sensitive Equipment. pp. 737–742 in Proceedings of the Inter-Noise Conference, Toronto, Ontario, Canada, . Vol. 1.
- USDA Forest Service. Wood Crate Design Manual. Agricultural Handbook Nº 252, .
Key Readings
- Allen, D.C. "Maximum Drops Experienced by Packages in Transit." See Symposium on Shock Environment of Packages in Transit. Journal of the Society of Environmental Engineers (), pp. 21–28.
- Brandenburg, R.K., and J. June-Ling Lee. Fundamentals of Packaging Dynamics. 4th edition. Michigan State University, School of Packaging. Skaneateles, NY: L.A.B., .
- Mecklenburg. M.F., ed. Art In Transit, Studies in the Transport of Paintings. Preprints for the International Conference on the Packing and Transportation of Paintings. Washington, DC: National Gallery of Art, , pp. 93–106 ("A Circular Slide Rule for Protective Package Design" by P. Marcon) and pp. 121–132 ("Shock, Vibration, and the Shipping Environment" by P. Marcon).
- Ostrem, F.E., and W.D. Godshall. An Assessment of the Common Carrier Shipping Environment. General Technical Report, Forest Products Laboratory, FPL 22, USDA. Madison, WI: U.S. Department of Agriculture, .
Thanks to the Centro Nacional de Conservación y Restauración in Chile, the Canadian Conservation Institute’s web resource Agents of deterioration, translated into Spanish by ICCROM, is now available free of charge. Intended for curators and conservators, the resource identifies 10 primary threats specific to heritage environments.
Page details
- Date modified: