Fifth Report on Human Biomonitoring of Environmental Chemicals in Canada
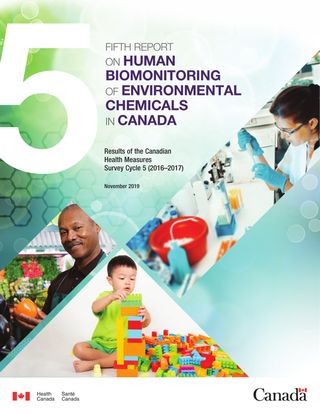
Download the alternative format
(PDF format, 7.3 MB, 436 pages)
Organization: Health Canada
Date published: 2019-11-13
Results of the Canadian Health Measures Survey Cycle 5 (2016-2017)
Table of Contents
- 1 Introduction
- 2 Objectives
- 3 Survey design
- 4 Fieldwork
- 5 Laboratory analyses
- 5.1 Metals and trace elements
- 5.1.1 Blood analyses
- 5.1.2 Urine analyses
- 5.2 Self-care and consumer product chemicals
- 5.2.1 Bisphenol A
- 5.2.2 Parabens
- 5.3 Cotinine
- 5.4 Acrylamide
- 5.5 Perfluoroalkyl and polyfluoroalkyl substances
- 5.6 Pesticides
- 5.6.1 Ethylene bisdithiocarbamates
- 5.6.2 ortho-Phenylphenol
- 5.6.3 Organophosphate pesticides
- 5.6.4 Pyrethroids
- 5.7 Plasticizers
- 5.8 Volatile organic compounds
- 5.9 Creatinine
- 5.1 Metals and trace elements
- 6 Statistical data analyses
- 7 Considerations for interpreting the biomonitoring data
- 8 Summaries and results for metals and trace elements
- 9 Summaries and results for self-care and consumer product chemicals
- 9.1 Bisphenol A
- 9.2 Parabens
- 10 Summary and results for nicotine
- 10.1 Nicotine
- 11 Summary and results for acrylamide
- 11.1 Acrylamide
- 12 Summary and results for perfluoroalkyl and polyfluoroalkyl substances
- 13 Summaries and results for pesticides
- 13.1 Organophosphate pesticides
- 13.2 Pyrethroids
- 13.3 Ethylene bisdithiocarbamates
- 13.4 ortho-Phenylphenol
- 14 Summaries and results for plasticizers
- 15 Summaries and results for volatile organic compounds
- 15.1 Benzene
- 15.2 Carbon tetrachloride
- 15.3 1,4-Dichlorobenzene
- 15.4 2,5-Dimethylfuran
- 15.5 Ethylbenzene
- 15.6 Isopropylbenzene
- 15.7 Methyl isobutyl ketone
- 15.8 Nitrobenzene
- 15.9 Styrene
- 15.10 1,1,1,2-Tetrachloroethane
- 15.11 Tetrachloroethylene
- 15.12 Tetrahydrofuran
- 15.13 Toluene
- 15.14 Trichloroethylene
- 15.15 Trihalomethanes
- 15.16 Xylenes
- Appendix A: Limits of detection
- Appendix B: Conversion factors
- Appendix C: Creatinine
- Appendix D: Biomonitoring of metals and trace elements in hair
Acknowledgements
This document was made possible by the efforts of the following staff of the National Biomonitoring Program of Health Canada: Annie St-Amand (Section Head), Jeff Willey (Report Lead), Kate Werry (Report Coordinator), Sarah Faure, Subramanian Karthikeyan, Pascale Lyonnais-Gagnon, Christine MacKinnon-Roy, Tyler Pollock, Julie Yome. With assistance from: Andrew Belknap, Kathy Nguyen, Eric Vaillancourt.
The development and implementation of the biomonitoring component of the Canadian Health Measures Survey was achieved through extensive contributions of programs and staff across Health Canada and Statistics Canada. A special thank you goes out to the participants of the survey, without whom this study would not be possible.
1 Introduction
These data tables present national data on concentrations of environmental chemicals in Canadians. These data were collected as part of an ongoing national direct health measures survey called the Canadian Health Measures Survey (CHMS). Statistics Canada, in partnership with Health Canada and the Public Health Agency of Canada, launched the CHMS in 2007 to collect health and wellness data and biological specimens on a nationally representative sample of Canadians. Biological specimens were analyzed for indicators of health status, chronic and infectious diseases, nutritional status, and environmental chemicals.
The CHMS biomonitoring component measures many environmental chemicals and/or their metabolites in the blood and urine of survey participants. Hair measures were also included for a subsample of participants in cycle 5. An environmental chemical can be defined as a chemical substance, either human-made or natural, that is present in the environment and to which humans may be exposed through media such as air, water, food, soil, dust, or consumer products.
Data from previous cycles have been published in four Health Canada reports, the most recent of which, the Fourth Report on Human Biomonitoring of Environmental Chemicals in Canada, was published in August 2017 (Health Canada, 2017). During the first four cycles, data were collected for 164 environmental chemicals in individual samples.
Data for cycle 5 were collected between January 2016 and December 2017 from approximately 5,800 Canadians aged 3–79 years at16 sites across Canada. Cycle 5 included 99 environmental chemicals, 64 of which were also measured in previous cycles.
A summary of the environmental chemicals measured in the blood and/or urine of individual respondents in the first five cycles of the CHMS is presented in Table 1.1.
Chemical group | Cycle 1 (2007–2009) |
Cycle 2 (2009–2011) |
Cycle 3 (2012–2013) |
Cycle 4 (2014–2015) |
Cycle 5 (2016–2017) |
---|---|---|---|---|---|
Flame retardants | Yes | No | No | No | No |
Organochlorines | Yes | No | No | No | No |
Polychlorinated biphenyls | Yes | No | No | No | No |
Chlorophenols | Yes | Yes | No | No | No |
Per- and polyfluoroalkyl substances | Yes | Yes | No | No | Yes |
Plasticizers | Yes | Yes | No | No | Yes |
Polycyclic aromatic hydrocarbons | No | Yes | Yes | Yes | No |
Acrylamide | No | No | Yes | Yes | Yes |
Volatile organic compounds | No | No | Yes | Yes | Yes |
Metals and trace elements | Yes | Yes | Yes | Yes | Yes |
Self-care and consumer product chemicals | Yes | Yes | Yes | Yes | Yes |
Nicotine | Yes | Yes | Yes | Yes | Yes |
Pesticides | Yes | Yes | Yes | Yes | Yes |
Collection for cycle 6 of the CHMS began in January 2018 and will be completed in late 2019. Planning for future cycles is under way.
This report describes the general CHMS survey design and implementation, with emphasis on the biomonitoring component. These sections are followed by descriptive summaries for each chemical, outlining the chemical's identity, common uses, occurrence in the environment, potential sources of exposure in the human population, toxicokinetics and health effects, Canadian regulatory status, and existing Canadian biomonitoring data.
Data tables specific to each chemical are provided below the relevant text; the tables are broken down by age group and sex, and contain descriptive statistics on the distribution of blood and/or urine concentrations in the sample population. For chemicals that were also measured in previous cycles, data from all cycles are presented together in tables for ease of comparison. Data for chemicals measured in hair can be found in Appendix D. For chemicals only measured in previous cycles, data can be found in previous reports (Health Canada, 2010; Health Canada, 2013; Health Canada, 2015; Health Canada, 2017). Downloadable tables are available in XLS format through the Government of Canada's Open data portal.
References
- Health Canada (2010). Report on Human Biomonitoring of Environmental Chemicals in Canada: Results of the Canadian Health Measures Survey Cycle 1 (2007–2009). Minister of Health, Ottawa, ON. Retrieved September 1, 2011.
- Health Canada (2013). Second Report on Human Biomonitoring of Environmental Chemicals in Canada: Results of the Canadian Health Measures Survey Cycle 2 (2009–2011). Minister of Health, Ottawa, ON. Retrieved May 30, 2013.
- Health Canada (2015). Third Report on Human Biomonitoring of Environmental Chemicals in Canada: Results of the Canadian Health Measures Survey Cycle 3 (2012–2013). Minister of Health, Ottawa, ON. Retrieved June 10, 2016.
- Health Canada (2017). Fourth Report on Human Biomonitoring of Environmental Chemicals in Canada: Results of the Canadian Health Measures Survey Cycle 4 (2014–2015). Minister of Health, Ottawa, ON. Retrieved October 19, 2018.
2 Objectives
The primary purpose of the biomonitoring component of the Canadian Health Measures Survey (CHMS) is to provide human biomonitoring data to scientists and health and environment officials to help them assess exposure to environmental chemicals and develop policies to reduce Canadians' exposure to toxic chemicals for the protection of their health.
Some specific uses of the CHMS biomonitoring data include:
- establishing baseline concentrations of chemicals in Canadians that allow for comparisons with subpopulations in Canada and with populations in other countries
- establishing baseline concentrations of chemicals to track trends in Canadians over time
- providing information for setting priorities and taking action to reduce Canadians' exposure to environmental chemicals and protect their health
- assessing the effectiveness of health and environmental risk management actions intended to reduce exposures and health risks from specific chemicals
- supporting future research on the potential links between exposure to certain chemicals and specific health effects
- contributing to international monitoring programs, such as the Stockholm Convention on Persistent Organic Pollutants
3 Survey design
The Canadian Health Measures Survey (CHMS) was designed as a cross-sectional survey to address important data gaps and limitations in existing health information in Canada. Its principal objective is to collect national-level baseline data on important indicators of Canadians' health status, including those pertaining to exposures to environmental chemicals. This information is important in understanding exposure risk factors, detecting emerging trends in risk factors and exposures, and advancing health surveillance and research in Canada. Detailed descriptions of the CHMS rationale, survey design, sampling strategy, and mobile examination centre (MEC) operations and logistics for cycle 5 have been published (Beck et al., 2018; Statistics Canada, 2019).
3.1 Target population
Cycle 5 of the CHMS targets the population aged 3–79 years living in one of the 10 provinces. The following groups were excluded from the survey: persons living in the three territories; persons living on reserves and other Indigenous settlements in the provinces; full-time members of the Canadian Forces; the institutionalized population; and residents of certain remote regions. Altogether, these exclusions represent approximately 3% of the target population.
Although the CHMS is not able to provide representative data for the entire Canadian population, there are a number of surveys and research projects carried out in partnership with Health Canada that directly target some of these population gaps.
The First Nations Biomonitoring Initiative (FNBI) is a survey carried out by the Assembly of First Nations (AFN) and Health Canada that seeks to establish baseline biomonitoring data for First Nations people living on-reserve south of the 60° parallel (AFN, 2013). Between 2009 and 2011, the FNBI measured the levels of 97 environmental chemicals in blood and urine samples collected from 503 participants living in 13 First Nations communities across Canada. The complete report has been published by the AFN.
In addition, numerous biomonitoring studies have been undertaken in Canada's North through the Northern Contaminants Program (NCP). The NCP, which is managed by federal government departments, provincial and territorial agencies, and Indigenous organizations, was established in 1991 to respond to concerns about human exposure to contaminants in the traditional diets of Northern Indigenous peoples. The NCP provides funding for numerous individual studies undertaken in various regions of the North, including the Northwest Territories, Nunavut, and Nunavik (Québec's North). More detailed information and results from these studies have been summarized in the Canadian Arctic Contaminants Assessment Reports and numerous scientific articles.
3.2 Sample size and allocation
To meet the objective of producing reliable estimates at the national level by age group and sex, cycle 5 of the CHMS required a minimum sample of at least 5,700 participants over a two-year period. The participants were distributed among age groups (3–5, 6–11, 12–19, 20–39, 40–59, and 60–79 years) and sex (except for 3–5 years), for a total of 11 groups. For the 3–5-year age group, the survey was not designed to provide estimates for the individual sexes.
3.3 Sampling strategy
To meet the requirements of the CHMS, a multistage sampling strategy was used.
3.3.1 Sampling of collection sites
The CHMS required participants to report to a MEC and be able to travel to it within a reasonable period of time. For cycle 5, Census geography was used to create 379 collection sites across the country. A geographic area with a population of at least 10,000 and a maximum participant travel distance of 50 km in urban areas and 75 km in rural areas was required for the location of collection sites. Areas not meeting these criteria were excluded.
Including a larger number of collection sites with few respondents would have optimized the precision of the estimates. However, the logistical and cost constraints associated with the use of MECs restricted the number of collection sites to 16. The 16 collection sites were selected from within the five standard regional boundaries used by Statistics Canada (the Atlantic provinces, Québec, Ontario, the Prairies, and British Columbia) and were allocated to these regions in proportion to population size. Although not every province in Canada had a collection site, the CHMS sites were chosen to represent the Canadian population in all 10 provinces, including larger and smaller population densities. The collection sites selected for cycle 5 of the CHMS are listed in Table 3.3.1.1.
Region | Collection Site |
---|---|
Atlantic |
|
Québec |
|
Ontario |
|
Prairies |
|
British Columbia |
|
3.3.2 Dwelling and participant sampling
Within each site, the most recent version of the Household Survey Frame, as well as more current information from other administrative sources, was used to select dwellings and identify the birth dates of household members. Dwellings with known household composition at the time of the sample selection were stratified by age of household residents at the time of the survey, with the six age-group strata corresponding to the CHMS cycle 5 age groups (3–5, 6–11, 12–19, 20–39, 40–59, and 60–79 years). Within each site, a simple random sample of dwellings was selected in each stratum. Each selected dwelling was then contacted and asked to provide a list of current household members; this list was used to select the survey participants. One or two people were selected, depending on the household composition.
3.4 Selection of environmental chemicals
A series of formal and informal consultations were carried out to determine the set of environmental chemicals measured in cycle 5 of the CHMS. The consultations included stakeholders with expertise or interest in human biomonitoring of environmental chemicals. Key participants were various internal Health Canada branches and programs as well as a number of external groups, including other federal departments, provincial/territorial health and environment departments, industry groups, environment and health non-governmental organizations, and academics.
The following criteria were used as general guides for identifying and selecting the environmental chemicals to include in the CHMS:
- seriousness of known or suspected health effects related to the substance
- need for public health actions related to the substance
- level of public concern about exposures and possible health effects related to the substance
- evidence of exposure of the Canadian population to the substance
- feasibility of collecting biological specimens in a national survey and associated burden on survey participants
- availability and efficiency of laboratory analytical methods
- costs of performing the test
- parity of selected chemicals with other national and international surveys and studies
- known data gaps
- commitments under national and international treaties, conventions, and agreements
- current and anticipated health policy development and implementation
- volume of biospecimens available from survey
A full list of the chemicals measured in the blood and/or urine of individual respondents in CHMS cycle 5 is presented in Table 3.4.1. Also included in this report are data for four organophosphate pesticide-related chemicals, namely 3,5,6-trichloro-2-pyridinol, malathion dicarboxylic acid, acephate, and methamidophos. While these chemicals were not measured in cycle 5, newly available data from cycle 3 are presented for them. Newly available data from cycle 3 for parabens are also presented. These data were not available for inclusion in previous reports due to delays in laboratory analyses.
Metals and trace elements were also measured in hair from a subsample of participants in cycle 5. Refer to Appendix D for a complete list of hair analytes.
Chemical | Cycle 1 (2007–2009) |
Cycle 2 (2009–2011) |
Cycle 3 (2012–2013) |
Cycle 4 (2014–2015) |
Cycle 5 (2016–2017) |
---|---|---|---|---|---|
Metals and trace elementsTable 3.4.1 footnote b | |||||
Lead | Yes | Yes | Yes | Yes | Yes |
Boron | No | No | No | No | Yes |
Cadmium | Yes | Yes | Yes | Yes | Yes |
Chromium (VI)Table 3.4.1 footnote c | No | No | No | No | Yes |
Selenium | Yes | Yes | No | No | Yes |
Arsenic (speciated) | |||||
Inorganic-related arsenic species | No | Yes | Yes | Yes | Yes |
Arsenite | No | Yes | Yes | Yes | Yes |
Arsenate | No | Yes | Yes | Yes | Yes |
Monomethylarsonic acid (MMA) | No | Yes | Yes | Yes | Yes |
Dimethylarsinic acid (DMA) | No | Yes | Yes | Yes | Yes |
Arsenocholine and arsenobetaine | No | Yes | Yes | Yes | Yes |
Mercury | |||||
Mercury (total) | Yes | Yes | Yes | Yes | Yes |
Methylmercury | No | No | Yes | Yes | Yes |
Mercury (inorganic) | Yes | No | Yes | Yes | Yes |
Self-care and consumer product chemicals | |||||
Bisphenol A (BPA) | Yes | Yes | Yes | Yes | Yes |
Parabens | |||||
Methyl paraben | No | No | YesTable 3.4.1 footnote d | Yes | Yes |
Ethyl paraben | No | No | YesTable 3.4.1 footnote d | Yes | Yes |
Propyl paraben | No | No | YesTable 3.4.1 footnote d | Yes | Yes |
Butyl paraben | No | No | YesTable 3.4.1 footnote d | Yes | Yes |
Nicotine | |||||
Cotinine | Yes | Yes | Yes | Yes | Yes |
Acrylamide | |||||
Acrylamide haemoglobin adduct | No | No | Yes | Yes | Yes |
Glycidamide haemoglobin adduct | No | No | Yes | Yes | Yes |
Per- and polyfluoroalkyl substances | |||||
Perfluorobutanoic acid (PFBA) | No | Yes | No | No | Yes |
Perfluorobutane sulfonate (PFBS) | No | Yes | No | No | Yes |
Perfluorohexanoic acid (PFHxA) | No | Yes | No | No | Yes |
Perfluorohexane sulfonate (PFHxS) | Yes | Yes | No | No | Yes |
Perfluorooctanoic acid (PFOA) | Yes | Yes | No | No | Yes |
Perfluorooctane sulfonate (PFOS) | Yes | Yes | No | No | Yes |
Perfluorononanoic acid (PFNA) | No | Yes | No | No | Yes |
Perfluorodecanoic acid (PFDA) | No | Yes | No | No | Yes |
Perfluoroundecanoic acid (PFUnDA) | No | Yes | No | No | Yes |
Pesticides | |||||
Organophosphate pesticides | |||||
Dimethylphosphate (DMP) | Yes | Yes | No | No | Yes |
Dimethylthiophosphate (DMTP) | Yes | Yes | No | No | Yes |
Dimethyldithiophosphate (DMDTP) | Yes | Yes | No | No | Yes |
Diethylphosphate (DEP) | Yes | Yes | No | No | Yes |
Diethylthiophosphate (DETP) | Yes | Yes | No | No | Yes |
Diethyldithiophosphate (DEDTP) | Yes | Yes | No | No | Yes |
3,5,6-Trichloro-2-pyridinol (TCPy) | No | No | YesTable 3.4.1 footnote d | Yes | No |
Malathion dicarboxylic acid (DCA) | No | No | YesTable 3.4.1 footnote d | Yes | No |
Acephate | No | No | YesTable 3.4.1 footnote d | No | No |
Methamidophos | No | No | YesTable 3.4.1 footnote d | No | No |
Ethylene bisdithiocarbamates | |||||
Ethylene thiourea (ETU) | No | No | No | No | Yes |
ortho-Phenylphenol (OPP) | |||||
OPP-glucuronide | No | No | No | No | Yes |
OPP-sulfate | No | No | No | No | Yes |
Pyrethroids | |||||
3-Phenoxybenzoic acid (3-PBA) | Yes | Yes | No | No | Yes |
4-Fluoro-3-phenoxybenzoic acid (4-F-3-PBA) | Yes | Yes | No | No | Yes |
cis-3-(2,2-Dibromovinyl)-2,2-dimethylcyclopropane carboxylic acid (cis-DBCA) | Yes | Yes | No | No | Yes |
cis-3-(2,2-Dichlorovinyl)-2,2-dimethylcyclopropane carboxylic acid (cis-DCCA) | Yes | Yes | No | No | Yes |
trans-3-(2,2-Dichlorovinyl)-2,2-dimethylcyclopropane carboxylic acid (trans-DCCA) | Yes | Yes | No | No | Yes |
Plasticizers | |||||
Monomethyl phthalate (MMP) | Yes | Yes | No | No | Yes |
Monoethyl phthalate (MEP) | Yes | Yes | No | No | Yes |
Mono(3-carboxypropyl) phthalate (MCPP) | Yes | Yes | No | No | Yes |
Mono-n-butyl phthalate (MnBP) | Yes | Yes | No | No | Yes |
Monoisobutyl phthalate (MiBP) | No | Yes | No | No | Yes |
Mono-3-hydroxy-n-butyl phthalate (3OH-MBP) | No | No | No | No | Yes |
Monocyclohexyl phthalate (MCHP) | Yes | Yes | No | No | Yes |
Monobenzyl phthalate (MBzP) | Yes | Yes | No | No | Yes |
Mono[2-(carboxymethyl)hexyl] phthalate (MCMHP) | No | No | No | No | Yes |
Mono(2-ethylhexyl) phthalate (MEHP) | Yes | Yes | No | No | Yes |
Mono(2-ethyl-5-carboxypentyl) phthalate (MECPP) | No | No | No | No | Yes |
Mono(2-ethyl-5-oxohexyl) phthalate (MEOHP) | Yes | Yes | No | No | Yes |
Mono(2-ethyl-5-hydroxyhexyl) phthalate (MEHHP) | Yes | Yes | No | No | Yes |
Mono-carboxy-n-heptyl phthalate (MCHpP) | No | No | No | No | Yes |
Mono-n-octyl phthalate (MOP) | Yes | Yes | No | No | Yes |
Mono(carboxyisooctyl) phthalate (MCiOP) | No | No | No | No | Yes |
Monoisononyl phthalate (MiNP) | Yes | Yes | No | No | Yes |
Monocarboxyisononyl phthalate (MCiNP) | No | No | No | No | Yes |
Monooxoisononyl phthalate (MOiNP) | No | No | No | No | Yes |
Monohydroxyisononyl phthalate (MHiNP) | No | No | No | No | Yes |
Monoisodecyl phthalate (MiDP) | No | No | No | No | Yes |
Monooxoisodecyl phthalate (MOiDP) | No | No | No | No | Yes |
Monohydroxyisodecyl phthalate (MHiDP) | No | No | No | No | Yes |
Di(isononyl)cyclohexane-1,2-dicarboxylate (DINCH) | |||||
trans-Cyclohexane-1,2-dicarboxylic mono isononyl ester (trans-MINCH) | No | No | No | No | Yes |
Cyclohexane-1,2-dicarboxylic mono oxo-isononyl ester (oxo-MINCH) | No | No | No | No | Yes |
Cyclohexane-1,2-dicarboxylic mono hydroxy-isononyl ester (OH-MINCH) | No | No | No | No | Yes |
cis-Cyclohexane-1,2-dicarboxylic mono carboxyisononyl ester (cis-cx-MINCH) | No | No | No | No | Yes |
trans-Cyclohexane-1,2-diarboxylic mono carboxyisononyl ester (trans-cx-MINCH) | No | No | No | No | Yes |
Cyclohexane-1,2-dicarboxylic acid (CHDA) | No | No | No | No | Yes |
2,2,4-Trimethyl-1,3-pentanediol diisobutyrate (TXIB) | |||||
2,2,4-Trimethyl-1,3-pentanediol (TMPD) | No | No | No | No | Yes |
2,2,4-Trimethyl-3-hydroxy valeric acid (HTMV) | No | No | No | No | Yes |
Tri-(2-ethylhexyl) trimellitate (TEHT) | |||||
1-Mono(2-ethylhexyl)trimellitate (1-MEHTM) | No | No | No | No | Yes |
2-Mono(2-ethylhexyl)trimellitate (2-MEHTM) | No | No | No | No | Yes |
4-Mono(2-ethylhexyl)trimellitate (4-MEHTM) | No | No | No | No | Yes |
Volatile organic compounds | |||||
Benzene | No | No | Yes | Yes | Yes |
Carbon tetrachloride | No | No | No | No | Yes |
1,4-Dichlorobenzene | No | No | No | No | Yes |
2,5-Dimethylfuran | No | No | No | No | Yes |
Ethylbenzene | No | No | Yes | Yes | Yes |
Isopropylbenzene | No | No | No | No | Yes |
Methyl isobutyl ketone | No | No | No | No | Yes |
Nitrobenzene | No | No | No | No | Yes |
Styrene | No | No | Yes | Yes | Yes |
1,1,1,2-Tetrachloroethane | No | No | No | No | Yes |
Tetrachloroethylene | No | No | Yes | Yes | Yes |
Tetrahydrofuran | No | No | No | No | Yes |
Toluene | No | No | Yes | Yes | Yes |
Trichloroethylene | No | No | Yes | Yes | Yes |
Trihalomethanes | |||||
Bromodichloromethane | No | No | Yes | Yes | Yes |
Dibromochloromethane | No | No | Yes | Yes | Yes |
Tribromomethane (bromoform) | No | No | Yes | Yes | Yes |
Trichloromethane (chloroform) | No | No | Yes | Yes | Yes |
Xylenes | |||||
m-Xylene and p-xylene | No | No | Yes | Yes | Yes |
o-Xylene | No | No | Yes | Yes | Yes |
Owing to the high cost of laboratory analyses, some environmental chemicals were not measured for all CHMS participants in cycle 5. The majority of the environmental chemicals were measured in a subsample of 2,500 participants aged 3–79 years, with the following exceptions: lead, cadmium, total mercury and selenium in blood, and cotinine in urine, were measured in all participants; methylmercury was measured in participants aged 3–19 years; volatile organic compounds were measured in 2,500 participants aged 12–79 years; and metals and trace elements in hair were measured in 2,000 participants aged 20–59 years. Further details on the subsampling for environmental chemicals are available in the Canadian Health Measures Survey (CHMS) Data User Guide: Cycle 5 (Statistics Canada, 2019) and in Sampling documentation for cycle 5 of the Canadian Health Measures Survey (Beck et al., 2018). For the chemicals measured in cycle 3 for which new data are presented in this report, further details on the subsampling for environmental chemicals are available in the Canadian Health Measures Survey (CHMS) Data User Guide: Cycle 3 (Statistics Canada, 2015) and in Sampling documentation for cycle 3 of the Canadian Health Measures Survey (Labrecque and Quigley, 2014).
Measure | Matrix | Target sample size | Age (years) | |||||
---|---|---|---|---|---|---|---|---|
3–5 | 6–11 | 12–19 | 20–39 | 40–59 | 60–79 | |||
Metals and trace elements | Blood | 5,700 | Yes | Yes | Yes | Yes | Yes | Yes |
Metals and trace elements | Hair | 2,000 | No | No | No | Yes | Yes | No |
Arsenic | Urine | 2,500 | Yes | Yes | Yes | Yes | Yes | Yes |
Cadmium and boron | Urine | 2,500 | Yes | Yes | Yes | Yes | Yes | Yes |
Chromium (VI)Table 3.4.2 footnote a | Red blood cells | 2,500 | Yes | Yes | Yes | Yes | Yes | Yes |
Methylmercury and inorganic mercury | Blood | 2,500 | Yes | Yes | Yes | No | No | No |
Bisphenol A (BPA) | Urine | 2,500 | Yes | Yes | Yes | Yes | Yes | Yes |
Parabens | Urine | 2,500 | Yes | Yes | Yes | Yes | Yes | Yes |
Cotinine | Urine | 5,700 | Yes | Yes | Yes | Yes | Yes | Yes |
Acrylamide | Blood | 2,500 | Yes | Yes | Yes | Yes | Yes | Yes |
Per- and polyfluoroalkyl substances | Plasma | 2,500 | Yes | Yes | Yes | Yes | Yes | Yes |
Organophosphate pesticides | Urine | 2,500 | Yes | Yes | Yes | Yes | Yes | Yes |
Pyrethroids | Urine | 2,500 | Yes | Yes | Yes | Yes | Yes | Yes |
Ethylene thiourea (ETU) | Urine | 2,500 | Yes | Yes | Yes | Yes | Yes | Yes |
ortho-Phenylphenol (OPP) | Urine | 2,500 | Yes | Yes | Yes | Yes | Yes | Yes |
Phthalates | Urine | 2,500 | Yes | Yes | Yes | Yes | Yes | Yes |
Di(isononyl)cyclohexane-1,2-dicarboxylate (DINCH) and tri-(2-ethylhexyl) trimellitate (TEHT) | Urine | 2,500 | Yes | Yes | Yes | Yes | Yes | Yes |
2,2,4-Trimethyl-1,3-pentanediol diisobutyrate (TXIB) | Urine | 2,500 | Yes | Yes | Yes | Yes | Yes | Yes |
Volatile organic compounds | Blood | 2,500 | No | No | Yes | Yes | Yes | Yes |
3.5 Ethical considerations
Personal information collected through the CHMS is protected under the federal Statistics Act (Canada, 1970-71-72). Under the Act, Statistics Canada is obliged to safeguard and keep in trust the information it obtains from the Canadian public. Consequently, Statistics Canada has established a comprehensive framework of policies, procedures, and practices to protect confidential information against loss, theft, unauthorized access, disclosure, copying, or use; this includes physical, organizational, and technological measures. The steps taken by Statistics Canada to safeguard the information collected in the CHMS have been described previously (Day et al., 2007).
Ethics approval for all components of the CHMS was obtained from the Health Canada and Public Health Agency of Canada Research Ethics Board. Informed written consent for the MEC portion of the CHMS was obtained from participants older than 14 years of age. For younger children, a parent or legal guardian provided written consent, and children aged 6–13 years of age provided assent. Participation in this survey was voluntary, and participants could opt out of any part of the survey at any time.
A strategy was developed to communicate results to survey participants with the advice and expert opinion of the CHMS Laboratory Advisory Committee, the Physician Advisory Committee, l'Institut national de santé publique du Québec (the reference laboratory performing some of the environmental chemical analyses), and Health Canada's Research Ethics Board (Day et al., 2007). For the environmental chemicals, only results for cadmium, lead and mercury were actively reported to participants from all sites. However, participants could receive all other test results upon request to Statistics Canada. More information on reporting to participants, including the ethical challenges encountered, can be found in Haines et al. (2011).
References
- AFN (Assembly of First Nations) (2013). First Nations Biomonitoring Initiative: National results (2011). Assembly of First Nations, Ottawa, ON. Retrieved July 29, 2013.
- Beck, K., Giroux, S., and Tremblay, M. (2018). Sampling documentation for cycle 5 of the Canadian Health Measures Survey. Statistics Canada (internal document).
- Canada (1970-71-72). Statistics Act. c. 15, s. 1. Retrieved August 7, 2012.
- Day, B., Langlois, R., Tremblay, M., and Knoppers, B. (2007). Canadian Health Measures Survey: Sampling strategy overview. Health Reports, Special Issue Supp. 18, 31–35.
- Haines, D.A., Arbuckle, T.E., Lye, E., Legrand, M., Fisher, M., Langlois, R., and Fraser, W. (2011). Reporting results of human biomonitoring of environmental chemicals to study participants: A comparison of approaches followed in two Canadian studies. Journal of Epidemiology and Community Health, 65(3), 191–198.
- Labrecque, F., and Quigley, A. (2014). Sampling documentation for cycle 3 of the Canadian Health Measures Survey. Statistics Canada (internal document).
- Statistics Canada (2015). Canadian Health Measures Survey (CHMS) Data User Guide: Cycle 3. Ottawa, ON. Available upon request (infostats@canada.ca).
- Statistics Canada (2019). Canadian Health Measures Survey (CHMS) Data User Guide: Cycle 5. Ottawa, ON. Available upon request (infostats@canada.ca).
4 Fieldwork
Fieldwork for the Canadian Health Measures Survey (CHMS) cycle 5 took place over a period of two years from January 2016 to December 2017. Data were collected sequentially at 16 sites across Canada. The sites were ordered to take into account seasonality by region and the temporal effect, subject to operational and logistical constraints.
Statistics Canada mailed advance letters and brochures to households that were selected as outlined in Section 3.3.2, Dwelling and Participant Sampling. The mailing informed potential participants that they would be contacted for the survey's data collection.
Data were collected from each consenting survey participant through a household personal interview, using a computer-assisted method, and through a visit to a mobile examination centre (MEC) for physical measures and biospecimen collection. The field team consisted of household interviewers and the CHMS MEC staff, including trained health professionals who performed the physical measures testing (Statistics Canada, 2019).
Participants were first administered a household questionnaire in their homes. Using a computer application, the interviewer randomly selected one or two participants and conducted separate 45- to 60-minute health interviews (Statistics Canada, 2019). The interviews collected demographic and socio-economic data, including information about lifestyle, medical history, current health status, smoking status and electronic cigarette use, and neighbourhood environment. Participants were also informed that Statistics Canada would link the information collected during the interview to information from the tax data of all members of their household. Within approximately two weeks of the home visit, participants visited the MEC. Each MEC consisted of three trailers linked by enclosed pedestrian walkways. One trailer was for reception and contained an administration area and an examination room; the second trailer contained a laboratory, a phlebotomy (blood collection) area, and examination rooms; and the third trailer contained additional examination rooms. The MEC operated for five to six weeks at each site to complete approximately 350 visits (Statistics Canada, 2019). MEC appointments averaged 2.5 hours. A parent or legal guardian accompanied children under 14 years of age. To maximize response rates, participants who were unable or unwilling to go to the MEC were offered the option of a home visit by CHMS MEC staff members to perform some of the physical measures and the biospecimen collection portion of the survey; there were seven home visits in total in cycle 5 (Statistics Canada, 2019).
At the start of the MEC visit, participants signed consent/assent forms prior to any testing, and in most cases provided a urine sample immediately thereafter. For logistical purposes, spot samples were collected rather than 24-hour urine samples. The urine samples were collected using first-catch urine. (To note, as an exception, mid-stream urine was collected in cycle 1.) Guidelines were provided to participants asking them to abstain from urinating two hours prior to their MEC visit. Samples were collected in 120 mL urine specimen containers.
Trained health professionals collected hair samples and took physical health measurements, such as for height, weight, blood pressure, and physical fitness. A series of screening questions were administered to participants to determine their eligibility for the various tests, including phlebotomy and hair sampling, based on pre-existing exclusion criteria (Statistics Canada, 2019). A minimum natural hair length of 2 cm was required; approximately 100 strands of full-length hair were collected from the back of the scalp. Blood specimens were drawn by a certified phlebotomist; the maximum amount depended upon the age of the participant and consent to storage. The approximate volume drawn with and without consent to storage from participants aged 3–5 years was 25.5 mL and 22.5 mL; 6–11 years, 40.0 mL and 34.0 mL; 12–13 years, 59.0 mL and 39.0 mL; 14–19 years, 77.0 mL and 49.0 mL; and 20–79 years, 83.0 mL and 53.0 mL.
Standardized operating procedures were developed for the collection of blood, urine and hair specimens, processing and aliquoting procedures, and the shipping of biospecimens to ensure adequate data quality and standardize data collection. All blood and urine specimens collected in the MEC were processed and aliquoted in the MEC. Blood and urine specimens were stored in the MEC in either the refrigerator or the freezer, depending on the test, while hair specimens were stored at room temperature. All specimens were stored as soon as processing was complete to maintain sample integrity. A four-hour time limit from the point of collection was set for blood samples to be processed and stored; however, for most samples, this was completed within two hours. Given specific pre-analytical requirements for chromium (VI) in red blood cells, a time limit of three hours was set for processing and storing the samples. Once a week, the specimens were shipped on dry ice or in monitored refrigerated conditions to the reference laboratory for analyses. A priority sequence for laboratory analyses was established in the event that an insufficient volume of biospecimen was collected for complete analyses of the environmental chemicals as well as for analyses of infectious diseases, nutritional status, and chronic diseases. Details on the matrix, collection tubes, and aliquot volumes are presented in Table 4.1 in order of testing priority.
Measure | Matrix | Collection tube (size and typeTable 4.1 footnote a) |
Optimal volume Table 4.1 footnote b |
---|---|---|---|
Volatile organic compounds (VOCs) | Whole blood | 5 mL baked grey top | 5 mL |
Per- and polyfluoroalkyl substances | Plasma | 4.0, 6.0, or 10 mLTable 4.1 footnote c lavender EDTA | 1.3 mL |
Chromium (VI) | Red blood cells | 4.0, 6.0, or 10 mLTable 4.1 footnote c lavender EDTA | 1.2 mL |
Cadmium and boron | Urine | 120 mL urine specimen container | 1.8 mL |
Specific gravity | 0.3 mL | ||
Arsenic (speciated) | 1.0 mL | ||
Acrylamide | Whole blood | 4.0, 6.0, or 10 mLTable 4.1 footnote c lavender EDTA |
1.0 or 1.5 mLTable 4.1 footnote d |
Metals and trace elements | 1.0 or 1.8 mLTable 4.1 footnote d | ||
Ethylene thiourea (ETU) | Urine | 120 mL urine specimen container | 1.0 mL |
ortho-Phenylphenol (OPP) | 1.8 mL | ||
Creatinine | 0.5 mL | ||
2,2,4-Trimethyl-1,3-pentanediol diisobutyrate (TXIB) | 1.8 mL | ||
Di(isononyl)cyclohexane-1,2-dicarboxylate (DINCH) and tri-(2-ethylhexyl) trimellitate (TEHT) | 2.0 mL | ||
Cotinine | 1.8 mL | ||
Phthalates | 2.5 mL | ||
Parabens | 1.0 mL | ||
Pyrethroids | 3.0 mL | ||
Bisphenol A (BPA) and organophosphate pesticides | 1.3 mL | ||
Metals and trace elements | Hair | Ziploc bag | 3 cm in length |
EDTA: ethylenediaminetetraacetic acid |
To maximize the reliability and validity of the data and reduce systematic bias, the CHMS developed quality assurance and quality control protocols for all aspects of the fieldwork. Quality assurance for the MEC covered staff selection and training, instructions to respondents (pre-testing guidelines), and issues related to data collection. All staff had appropriate education and training for their respective positions. To ensure consistent measurement techniques, procedure manuals and training guides were developed in consultation with, and reviewed by, experts in the field. Quality control samples were evaluated for each site and consisted of field blanks, blind replicates, and blind controls. Three field blanks (deionized water) were analyzed per site for all analytes except acrylamide and VOCs in blood, and creatinine and cotinine in urine. Three pairs of blind replicates were assessed per site for all analytes. Approximately six blind control samples were evaluated per site for all analytes except chromium (VI) in red blood cells.
Field blanks were sent to the reference laboratories at the start of each site and results were expeditiously returned directly to the laboratory coordinators at Statistics Canada. Blind replicate and blind control samples were sent to the reference laboratories with regular specimen shipments. Quality control sample results were sent to Statistics Canada's CHMS headquarters, along with all other respondent results. If required, feedback was provided quickly to the relevant reference laboratory for review and remedial action.
Detailed descriptions of the CHMS MEC operations and logistics have been described previously in Bryan et al. (2007) and are presented in the Canadian Health Measures Survey (CHMS) Data User Guide: Cycle 5 (Statistics Canada, 2019).
References
- Bryan, S.N., St-Denis, M., and Wojitas, D. (2007). Canadian Health Measures Survey: Operations and logistics. Health Reports, Special Issue Supp. 18, 53–70.
- Statistics Canada (2019). Canadian Health Measures Survey (CHMS) Data User Guide: Cycle 5. Ottawa, ON. Available upon request (infostats@canada.ca).
5 Laboratory analyses
Laboratory analyses of environmental chemicals and creatinine were performed at analytical laboratories within Health Canada and at l'Institut national de santé publique du Québec (INSPQ). Laboratories developed standardized operating procedures for the analytical methods used to measure environmental chemicals or their metabolites in biological samples. Analytical accuracy and the precision of measurements were evaluated through rigorous method validation programs at each laboratory.
To ensure ongoing accuracy and precision of results, several quality control measures were employed as part of the Canadian Health Measures Survey (CHMS). Field blanks were used to confirm that samples had not been contaminated during collection, processing, storage, or shipping. Blind replicate samples were used as indicators of the precision of sample analysis, while blind control samples were used as indicators of the accuracy of sample analysis. Laboratories also participated in external quality control programs and interlaboratory comparison studies, as outlined in the sections below. The methods used in the analyses of the environmental chemicals and creatinine are described below.
5.1 Metals and trace elements
5.1.1 Blood analyses
5.1.1.1 Lead, cadmium, selenium, and total mercury
Lead, cadmium, selenium, and mercury analyses in whole blood were performed at the Centre de toxicologie du Québec (CTQ), INSPQ (INSPQ, 2018p). Briefly, whole blood samples were diluted in a basic solution containing octylphenol ethoxylate and ammonium hydroxide and analyzed for lead, cadmium, selenium, and mercury using inductively coupled plasma mass spectrometry (ICP-MS). The ICP-MS method employed a Perkin Elmer Sciex Elan DRC II with an ESI SC-4 autosampler and an Elan workstation version 3.0. Matrix-matched calibration was performed using blood from non-exposed individuals. Internal quality control was ensured by analyzing two different reference materials from the Québec Multielement External Quality Assessment Scheme (QMEQAS) in each analysis sequence. The external quality and accuracy of the analytical method were assessed by participating in interlaboratory comparison programs, including the internal CTQ Programme de comparaisons interlaboratoires pour les métaux en milieu biologique (PCI); QMEQAS; the German External Quality Assessment Scheme (G-EQUAS); the U.S. Centers for Disease Control and Prevention's Lead and Multielement Proficiency Program (LAMP); and the New York State Department of Health's Proficiency Program for Trace Elements in Whole Blood.
5.1.1.2 Chromium (VI)
Chromium (VI) analyses in red blood cells were performed at the CTQ, INSPQ(INSPQ, 2018f). The analysis was an indirect measurement of chromium (VI) and was based on the fact that chromium (VI) is the only form of inorganic chromium to penetrate cells. As such, chromium measured in red blood cells is attributed specifically to chromium (VI) exposure (Devoy et al., 2016).
Briefly, red blood cells were purified shortly after collection via a saline wash. Purified red blood cells were digested with concentrated nitric acid and hydrogen peroxide and diluted in water to reduce viscosity. The samples were then analyzed using inductively coupled plasma tandem mass spectrometry (ICP-MS-MS). The ICP-MS-MS method employed an Agilent Technologies 8800 ICP-QQQ with a CETAC ASX-500 autosampler and a MassHunter 4.2 workstation version C.01.02. Terbium was used as an internal standard. Internal quality control was ensured by analyzing three different in-house reference materials (low, medium and high) in each analysis sequence.
5.1.1.3 Methylmercury and inorganic mercury
Methylmercury and inorganic mercury analyses in whole blood were performed at the CTQ, INSPQ (INSPQ, 2018k). Briefly, whole blood samples were digested with tetramethylammonium hydroxide and mercury species were derivatized into volatile compounds by sodium tetra-n-propylborate. Mercury was extracted in the gas phase by solid-phase microextraction with polydimethylsiloxane/divinylbenzene fibre. Ultimately, mercury species were analyzed using isotopic dilution in tandem gas chromatography and inductively coupled plasma mass spectrometry (ID-GC-ICP-MS). The ID-GC-ICP-MS method employed a Perkin Elmer Clarus 580 gas chromatograph with a Zebron ZB-5 column (Phenomenex), a CTC Analytics CombiPAL autosampler, and an Empower chromatograph workstation version 3 alongside a Perkin Elmer NexION 350s ICP-MS with a Syngistix workstation version 1.1. Quantification was obtained by isotope dilution calculation. Internal quality control was ensured by analyzing three different in-house reference materials (low, medium, and high) in each analysis sequence.
5.1.2 Urine analyses
5.1.2.1 Arsenic
Speciated arsenic analyses in urine were performed at the CTQ, INSPQ (INSPQ, 2018j). The analyses measured arsenite (As3+), arsenate (As5+), monomethylarsonic acid, dimethylarsinic acid, and the sum of arsenobetaine and arsenocholine. Briefly, urine samples were diluted tenfold in an ammonium carbonate solution (dilution solvent) compatible with the initial eluent, then analyzed on the high-performance liquid chromatography system, used in high pressure mode only, combined with inductively coupled plasma mass spectrometry (HPLC-ICP-MS). The HPLC-ICP-MS method employed a Waters ACQUITY HPLC with an Empower chromatograph workstation version 3 and a Perkin Elmer NexION 350s ICP-MS with a Syngistix workstation version 1.1. Methylseleno-L-cysteine was used as an internal standard. Internal quality control was ensured by analyzing three non-certified, in-house reference materials in each analysis sequence. External quality and the accuracy of the analytical method were assessed by participating in interlaboratory comparison programs, including the G-EQUAS.
5.1.2.2 Boron
Boron analyses in urine were performed at the CTQ, INSPQ (INSPQ, 2018e). Briefly, urine samples were diluted in 0.5% nitric acid and analyzed for boron using ICP-MS-MS. The ICP-MS-MS method employed an Agilent Technologies 8800 ICP-QQQ with a CETAC ASX-500 autosampler and a MassHunter 4.2 workstation version C.01.02. Beryllium was used as an internal standard. Internal quality control was ensured by analyzing three different in-house reference materials (low, medium, and high) in each analysis sequence.
5.1.2.3 Cadmium
Cadmium analyses in urine were performed at the CTQ, INSPQ (INSPQ, 2018q). Briefly, urine samples were diluted in 0.5% nitric acid and analyzed for cadmium using ICP-MS. The ICP-MS method employed a Perkin Elmer Sciex Elan DRC II with an ESI SC-4 autosampler and an Elan workstation version 3.0. Matrix-matched calibration was performed using urine from non-exposed individuals. Correction of molybdenum-based interference on cadmium concentrations was performed mathematically using equations derived following the addition of molybdenum to urine samples. Internal quality control was ensured by analyzing three different reference materials from the QMEQAS in each analysis sequence. The external quality and accuracy of the analytical method were assessed by participating in interlaboratory comparison programs, including the internal CTQ PCI, QMEQAS, the G-EQUAS, and the New York State Department of Health's Proficiency Program for Trace Elements in Urine.
5.2 Self-care and consumer product chemicals
5.2.1 Bisphenol A
Bisphenol A analyses in urine were performed at the CTQ, INSPQ (INSPQ, 2018d). Briefly, urine samples were hydrolyzed using β -glucuronidase and derivatized with pentafluorobenzyl bromide. The derivatized products were then extracted with a mixture of dichloromethane and hexane. Extracts were then evaporated and redissolved, and the sum of free and conjugated forms of bisphenol A was analyzed by gas chromatography coupled with tandem mass spectrometry (GC-MS-MS). The GC-MS-MS method employed an Agilent 6890 gas chromatograph with an Agilent 7683 automatic injector and sampler coupled to a Waters Quattro Micro-GC tandem quadrupole mass spectrometer and a workstation equipped with Waters MassLynx software version 4.1; measurements were carried out in multiple reaction monitoring (MRM) mode with a source in negative chemical ionization mode. Carbon-13–labelled bisphenol A analogues were used as internal standards. Internal quality control was ensured by analyzing three different in-house reference materials (low, medium, and high) in each analysis sequence. The external quality and accuracy of the analytical method were assessed by participating in interlaboratory comparison programs, including the G-EQUAS.
5.2.2 Parabens
Paraben analyses in urine were performed at the Food Program Western Region Laboratory, Health Canada, British Columbia, Canada (Health Canada, 2017) using a method adapted from the U.S. Centers for Disease Control and Prevention (CDC, 2011). In these analyses, free and conjugated forms of butyl paraben, ethyl paraben, methyl paraben, and propyl paraben were measured together. Briefly, urine samples were hydrolyzed using β-glucuronidase/sulfatase (Helix pomatia type H1). After enzymatic hydrolysis, samples were acidified with formic acid and preconcentrated using solid-phase extraction (Waters Oasis HLB SPE tubes). The sum of free and conjugated parabens was detected and quantified using ultra-performance liquid chromatography coupled with tandem mass spectrometry (UPLC-MS-MS). The UPLC-MS-MS method employed a Waters ACQUITY UPLC coupled to a Waters Quattro Premier XE tandem mass spectrometer; data were collected as MRM data in electrospray ionization negative mode. Deuterated parabens (D4-methyl paraben, D4-ethyl paraben, D4-propyl paraben, and D4-butyl paraben) were used as the internal standards. Internal quality control was ensured by analyzing two in-house quality control pools (low and high) in each batch of analyses.
5.3 Cotinine
Free cotinine analyses in urine were performed at the CTQ, INSPQ. One method was used for participants aged 3–11 years (INSPQ, 2018a) and another for participants aged 12–79 years (INSPQ, 2018c). Data from the two methods were combined and are presented separately for smokers aged 12–79 years and non-smokers aged 3–79 years. Briefly, for both methods, free cotinine was extracted from urine samples by solid-phase extraction via mixed cation-exchange and reverse phase support on an automated Perkin Elmer JANUS automated liquid-handling workstation. The extracts were redissolved in the mobile phase and analyzed by UPLC-MS-MS. The UPLC-MS-MS method employed a Waters ACQUITY UPLC coupled to a Waters Xevo TQ-S or Quattro Premier XE tandem mass spectrometer and a workstation equipped with Waters MassLynx software version 4.1; measurements were carried out in MRM mode with an electrospray source positive mode. For participants aged 12–79 years, within each analysis sequence, samples from non-smokers were analyzed first, followed by samples from smokers, to avoid contamination between samples. Deuterated cotinine was used as an internal standard. Internal quality control was ensured by analyzing three different in-house reference materials (low, medium, and high) in each analysis sequence. The external quality and accuracy of the analytical method were assessed by participating in interlaboratory comparison programs, including the G-EQUAS.
5.4 Acrylamide
Acrylamide and glycidamide hemoglobin adduct analyses in whole blood were performed at the Ontario Food Laboratory, Health Canada, Ontario, Canada (Health Canada, 2014). Briefly, whole blood samples were reacted with modified Edman reagent (pentafluorophenyl isothiocyanate) and purified using solid-phase extraction on a column of ISOLUTE HM-N sorbent with a diisopropyl ether/ethyl acetate/toluene (50/40/10 v/v/v) eluent. The extract was evaporated, reconstituted, and analyzed using UPLC-MS-MS. The UPLC-MS-MS method employed a Waters ACQUITY ultra-performance liquid chromatograph system coupled to a Waters Quattro Premier tandem mass spectrometer and a workstation equipped with MassLynx software; measurements were carried out in MRM mode with an Atmospheric Pressure Chemical Ionization (APCI) positive ion mode. Carbon-13 labelled acrylamide octapeptide was used as an internal standard. Internal quality control was ensured by analyzing two different in-house reference materials (low and high) in each analysis sequence. Hemoglobin was also measured in whole blood using a commercial HemoCue assay kit; the hemoglobin value was used to adjust the acrylamide and glycidamide hemoglobin adduct results.
5.5 Perfluoroalkyl and polyfluoroalkyl substances
Perfluoroalkyl substance analyses in plasma were performed at the CTQ, INSPQ (INSPQ, 2018i). The analyses measured perfluorobutanoic acid, perfluorobutane sulfonate, perfluorohexanoic acid (PFHxA), perfluorohexane sulfonate (PFHxS), perfluorooctane sulfonate (PFOS), perfluorooctanoic acid (PFOA), perfluorononanoic acid (PFNA), perfluorodecanoic acid (PFDA), and perfluoroundecanoic acid (PFUnDA). Briefly, plasma samples were extracted by solid-phase extraction with a WAX support on an automated Perkin Elmer JANUS automated liquid-handling workstation. The extracts were redissolved in the mobile phase and analyzed by UPLC-MS-MS. The UPLC-MS-MS method employed a Waters ACQUITY UPLC coupled to a Waters Xevo TQ-S tandem mass spectrometer and a workstation equipped with MassLynx software version 4.1; measurements were carried out in MRM mode with an electrospray ionization negative mode. Internal quality control was ensured by analyzing four different reference materials — three in-house (low, medium, and high) and one commercial — in each analysis sequence. External quality and accuracy of the analytical method was assessed by participating in interlaboratory comparison programs, including the internal CTQ Arctic Monitoring and Assessment Program (AMAP) ring test interlaboratory comparison program for persistent organic pollutants in human serum (PFHxA, PFHxS, PFNA, PFOA, PFOS, PFDA, PFUnDA) and the G-EQUAS for PFOS and PFOA.
5.6 Pesticides
5.6.1 Ethylene bisdithiocarbamates
Ethylene thiourea (ETU) analyses in urine were performed at the CTQ, INSPQ (INSPQ, 2018g). Briefly, urine samples were hydrolyzed and derivatized with 2,3,4,5,6-pentafluorobenzyl bromide. The derivatized products were then extracted with hexane. The extracts were analyzed by UPLC-MS-MS. The UPLC-MS-MS method employed a Waters ACQUITY UPLC coupled to a Waters Xevo TQ-S tandem mass spectrometer and a workstation equipped with MassLynx software version 4.1; measurements were carried out in MRM mode with electrospray ionization positive mode. Deuterated ETU was used as an internal standard. Internal quality control was ensured by analyzing four different reference materials — three in-house (low, medium, and high) and one commercial — in each analysis sequence.
5.6.2 ortho-Phenylphenol
ortho-Phenylphenol analyses in urine were performed at the CTQ, INSPQ (INSPQ, 2018r). In these analyses, glucuronide- and sulfate-conjugated forms of ortho-phenylphenol were measured. Briefly, urine samples were extracted on an ion-exchange cartridge, eluted, and evaporated to dryness. The extracts were redissolved in a mixture of methanol and demineralized water (25:75), then analyzed by UPLC-MS-MS. The UPLC-MS-MS method employed a Waters ACQUITY UPLC coupled to a Waters Xevo TQ-S tandem mass spectrometer and a workstation equipped with MassLynx software version 4.1; measurements were carried out in MRM mode with an electrospray ionization negative mode. Carbon-13 labelled ortho-phenylphenol was used as an internal standard. Internal quality control was ensured by analyzing three different in-house reference materials (low, medium, and high) in each analysis sequence.
5.6.3 Organophosphate pesticides
5.6.3.1 Dialkyl phosphates
Dialkyl phosphate metabolite analyses in urine were performed at the CTQ, INSPQ (INSPQ, 2018h). The analyses measured dimethylphosphate (DMP), dimethylthiophosphate (DMTP), dimethyldithiophosphate (DMDTP), diethylphosphate (DEP), diethylthiophosphate (DETP), and diethyldithiophosphate (DEDTP). Briefly, urine samples were hydrolyzed using β-glucuronidase and derivatized with pentafluorobenzyl bromide. The derivatized products were then extracted with a mixture of dichloromethane and hexane. Extracts were redissolved and analyzed by GC-MS-MS. The GC-MS-MS method employed an Agilent 6890 gas chromatograph with an Agilent 7683 automatic injector and sampler coupled to a Waters Quattro Micro-GC tandem quadrupole mass spectrometer and a workstation equipped with Waters MassLynx software version 4.1; measurements were carried out in MRM mode with a source in negative chemical ionization mode. Isotopically labelled dialkyl phosphate metabolite analogues were used as internal standards. Internal quality control was ensured by analyzing four different reference materials — three in-house (low, medium, and high) and one commercial — in each analysis sequence. External quality and accuracy of the analytical method was assessed by participating in interlaboratory comparison programs, including the G-EQUAS.
5.6.3.2 3,5,6-Trichloro-2-pyridinol
3,5,6-Trichloro-2-pyridinol (TCPy) analyses in urine were performed at the CTQ, INSPQ (INSPQ, 2015c). In these analyses, free and conjugated forms of TCPy were measured together. Briefly, urine samples were hydrolyzed with β-glucuronidase/arylsulfatase and derivatized with dansyl chloride. The derivatized products were then extracted with hexane. The extracts were redissolved in a mixture of acetonitrile, methanol, and water, then analyzed by UPLC-MS-MS. The UPLC-MS-MS method employed a Waters ACQUITY UPLC coupled to a Waters Xevo TQ-S tandem mass spectrometer and a workstation equipped with MassLynx software version 4.1; measurements were carried out in MRM mode with an electrospray ionization positive mode. Carbon-13 labelled TCPy was used as an internal standard. Internal quality control was ensured by analyzing three different in-house reference materials (low, medium, and high) in each analysis sequence. The external quality and accuracy of the analytical method were assessed by participating in interlaboratory comparison programs, including the G-EQUAS.
5.6.3.3 Malathion dicarboxylic acid
Malathion dicarboxylic acid (DCA) analyses in urine were performed at the CTQ, INSPQ (INSPQ, 2015b). Briefly, urine samples were extracted on an ion-exchange cartridge, eluted, and evaporated to dryness. Extracts were redissolved in ethyl acetate, derivatized with N-tert-butyldimethylsilyl-N-methyltrifluoroacetamide (MTBSTFA) and analyzed by gas chromatography coupled with tandem mass spectrometry (GC-MS-MS). The GC-MS-MS method employed an Agilent 7890A gas chromatograph with an Agilent 7693 automatic injector and sampler coupled to an Agilent 7000B tandem mass spectrometer and a workstation equipped with Waters MassHunter software; measurements were carried out in MRM mode with a source in electron ionization (EI) mode. Carbon-13 labelled DCA was used as an internal standard. Internal quality control was ensured by analyzing three different in-house reference materials (low, medium, and high) in each analysis sequence.
5.6.3.4 Acephate and methamidophos
Acephate and methamidophos analyses in urine were performed at the CTQ, INSPQ (INSPQ, 2015a). Briefly, urine samples were extracted with dichloromethane under acidic conditions. The extracts were analyzed by UPLC-MS-MS. The UPLC-MS-MS method employed a Waters ACQUITY UPLC coupled to a Waters Xevo TQ-S tandem mass spectrometer and a workstation equipped with MassLynx software version 4.1; measurements were carried out in MRM mode with an electrospray ionization positive mode. Internal quality control was ensured by analyzing three different in-house reference materials (low, medium, and high) in each analysis sequence.
5.6.4 Pyrethroids
Pyrethroid analyses in urine were performed at the CTQ, INSPQ (INSPQ, 2018m). The analyses measured 3-phenoxybenzoic acid (3-PBA), 4-fluoro-3-phenoxybenzoic acid (4-F-3-PBA), cis-3-(2,2-dibromovinyl)-2,2-dimethylcyclopropane carboxylic acid (cis-DBCA), cis-3-(2,2-dichlorovinyl)-2,2-dimethylcyclopropane carboxylic acid (cis-DCCA), and trans-3-(2,2-dichlorovinyl)-2,2-dimethylcyclopropane carboxylic acid (trans-DCCA). Briefly, urine samples were hydrolyzed using β-glucuronidase and then acidified and extracted with hexane. The extracts were derivatized with hexafluoro-2-propanol (HFIP) and diisopropylcarbodiimide (DIC), and re-extracted with hexane. Extracts were then analyzed by GC-MS. The GC-MS method employed an Agilent 6890 network gas chromatograph with an Agilent 7683B automatic injector and sampler coupled to an Agilent 5975 mass spectrometer and a workstation equipped with Waters MassHunter software version B.07.01 build 7.1.524.0 and ChemStation G1701EA software version E02.01.1177; measurements were carried out in single ion monitoring (SIM) modes following negative chemical ionization. Carbon-13 labelled trans-DCCA, 4-F-3-PBA, and 3-PBA analogues were used as internal standards; the isotopically labelled trans-DCCA analogue was used as an internal standard for cis-DCCA, trans-DCCA, and cis-DCBA. Internal quality control was ensured by analyzing four different reference materials, three in-house (low, medium, and high) and one commercial, in each analysis sequence. External quality and accuracy of the analytical method was assessed by participating in interlaboratory comparison programs, including the G-EQUAS, for cis-DBCA, cis-DCCA, trans-DCCA, and 3-PBA.
5.7 Plasticizers
5.7.1 Phthalates
Phthalate metabolite analyses in urine were performed at the CTQ, INSPQ (INSPQ, 2018l). The analyses measured 23 phthalate metabolites (see Table 3.4.1 for complete analyte list). Briefly, urine samples were hydrolyzed using β-glucuronidase and the analytes were extracted using liquid–liquid extraction with a hexane:ethyl acetate solution (50:50) on an automated Perkin Elmer JANUS automated liquid-handling workstation. The extracts were analyzed by UPLC-MS-MS. The UPLC-MS-MS method employed a Waters ACQUITY UPLC coupled to a Waters Xevo TQ-S tandem mass spectrometer and a workstation equipped with MassLynx software version 4.1; measurements were carried out in MRM mode with an electrospray ionization negative mode. Various internal standards were used including deuterated monoisobutyl phthalate (MiBP) and carbon-13 labelled monobenzyl phthalate (MBzP), monocyclohexyl phthalate (MCHP), monoisononyl phthalate (MiNP), monoethyl phthalate (MEP), monomethyl phthalate (MMP), mono-n-butyl phthalate (MnBP), mono-n-octyl phthalate (MOP), mono(2-ethylhexyl) phthalate (MEHP), mono(2-ethyl-5-hydroxyhexyl) phthalate (MEHHP), mono(2-ethyl-5-oxohexyl) phthalate (MEOHP), mono(3-carboxypropyl) phthalate (MCPP), mono(2-ethyl-5-carboxypentyl) phthalate (MECPP) analogues and deuterated mono[2-(carboxymethyl)hexyl] phthalate (MCMHP), monoisodecyl phthalate (MiDP), and mono-3-hydroxy-n-butyl phthalate
(3OH-MBP). In addition to MEHHP, the isotopically labelled MEHHP was used as an internal standard for mono-carboxy-n-heptyl phthalate (MCHpP), monocarboxyisononyl phthalate (MCiNP), mono(carboxyisooctyl) phthalate (MCiOP), monohydroxyisodecyl phthalate (MHiDP), monohydroxyisononyl phthalate (MHiNP), monooxoisodecyl phthalate (MOiDP) and monooxoisononyl phthalate (MOiNP). Internal quality control was ensured by analyzing three different in-house reference materials (low, medium, and high) in each analysis sequence. External quality and accuracy of the analytical method was assessed by participating in interlaboratory comparison programs including the G-EQUAS, for MEHHP, MEOHP, MECPP, MEHP, MnBP, MiBP, and MBzP.
Due to issues during the peak integration process, results were reported for MCiOP, MiNP and MCiNP semi-quantitatively. Results for all other analytes were reported quantitatively.
5.7.2 Di(isononyl)cyclohexane-1,2-dicarboxylate (DINCH) and tri-(2-ethylhexyl) trimellitate (TEHT)
Di(isononyl)cyclohexane-1,2-dicarboxylate (DINCH) and tri-(2-ethylhexyl) trimellitate (TEHT) metabolite analyses in urine were performed at the CTQ, INSPQ (INSPQ, 2018n). The analyses measured the DINCH metabolites trans-cyclohexane-1,2-dicarboxylic mono isononyl ester (trans-MINCH), cyclohexane-1,2-dicarboxylic mono oxoisononyl ester (oxo-MINCH), cyclohexane-1,2-dicarboxylic mono hydroxyisononyl ester (OH-MINCH), cis-cyclohexane-1,2-dicarboxylic mono carboxyisononyl ester (cis-cx-MINCH), trans-cyclohexane-1,2-diarboxylic mono carboxyisononyl ester (trans-cx-MINCH). The analyses also measured the TEHT metabolites 1-mono(2-ethylhexyl)trimellitate (1-MEHTM), 2-mono(2-ethylhexyl)trimellitate (2-MEHTM), and 4-mono(2-ethylhexyl)trimellitate (4-MEHTM). Briefly, urine samples were hydrolyzed using β-glucuronidase and the analytes were extracted using liquid–liquid extraction with a hexane:ethyl acetate solution (50:50) on an automated Perkin Elmer JANUS automated liquid-handling workstation. The extracts were taken up with a mixture of acetonitrile and demineralized water and analyzed by UPLC-MS-MS. The UPLC-MS-MS method employed a Waters ACQUITY UPLC coupled to a Waters Xevo TQ-S tandem mass spectrometer and a workstation equipped with MassLynx software version 4.1; measurements were carried out in MRM mode with an electrospray ionization-negative mode. Deuterated trans-cx-MINCH was used as an internal standard for trans-cx-MINCH, cis-cx-MINCH, oxo-MINCH, 1-MEHTM, 2-MEHTM, and 4-MEHTM. Deuterated trans-cyclohexane-1,2-dicarboxylic mono hydroxyisononyl ester (trans-OH-MINCH) was used as an internal standard for OH-MINCH and deuterated trans-MINCH was used as an internal standard for trans-MINCH. Internal quality control was ensured by analyzing three different in-house reference materials (low, medium, and high) in each analysis sequence.
5.7.3 2,2,4-Trimethyl-1,3-pentanediol diisobutyrate (TXIB) and cyclohexane-1,2-dicarboxylic acid (CHDA)
Cyclohexane-1,2-dicarboxylic acid (CHDA) and 2,2,4-trimethyl-1,3-pentanediol diisobutyrate (TXIB) metabolite analyses in urine were performed at the CTQ, INSPQ (INSPQ, 2018o). The analyses measured the di(isononyl)cyclohexane-1,2-dicarboxylate (DINCH) metabolite CHDA and the TXIB metabolites 2,2,4-trimethyl-1,3-pentanediol (TMPD) and 2,2,4-trimethyl-3-hydroxy valeric acid (HTMV). Briefly, urine samples were hydrolyzed using β-glucuronidase and arylsulfatase, acidified and extracted with ethyl acetate on an automated Perkin Elmer JANUS automated liquid-handling workstation. The extracts were taken up with a mixture of methanol and water and analyzed by UPLC-MS-MS. The UPLC-MS-MS method employed a Waters ACQUITY UPLC coupled to a Waters Xevo TQ-S tandem mass spectrometer and a workstation equipped with MassLynx software version 4.1; measurements were carried out in MRM mode with an electrospray ionization negative mode for HTMV and CHDA and positive mode for TMPD. Deuterated 2,2-bis(hydroxymethyl)pentane was used as an internal standard for TMPD. Deuterated HTMV and CHDA analogues were used as internal standards for HTMV and CHDA, respectively. Internal quality control was ensured by analyzing three different in-house reference materials (low, medium, and high) in each analysis sequence.
5.8 Volatile organic compounds
Volatile organic compound (VOC) analyses in blood were performed at the Exposure and Biomonitoring Division Laboratory, Health Canada (Aranda-Rodriguez et al., 2015; Health Canada, 2018). The analyses measured benzene, carbon tetrachloride, 1,4-dichlorobenzene, 2,5-dimethylfuran, ethylbenzene, isopropylbenzene, methyl isobutyl ketone, nitrobenzene, styrene, 1,1,1,2-tetrachloroethane, tetrachloroethylene, tetrahydrofuran, toluene, trichloroethylene, bromodichloromethane, dibromochloromethane, tribromomethane, trichloromethane, m-xylene, p-xylene, and o-xylene. Briefly, blood samples were extracted by solid-phase microextraction, focused in a cryotrap and analyzed by GC-MS-MS. The method employed a Thermo Fisher Scientific 915 cryotrap, TRACE™ ultra GC coupled to a TSQ Quantum XLS mass spectrometer and a workstation equipped with Xcalibur software version 3.1; measurements were carried out in selected reaction monitoring mode with an electron ionization source. Carbon-13 or deuterated analogues of the VOC analytes were used as internal standards. Internal quality control was ensured by analyzing three different in-house reference materials (low, medium, and high) in duplicate for each batch of analysis.
5.9 Creatinine
Creatinine analyses in urine were performed at the CTQ, INSPQ (INSPQ, 2018b) using the colorimetric end point Jaffe method. Briefly, urine samples were reacted with an alkaline solution of sodium picrate to form a red Janovski complex. The complex was analyzed by spectrophotometry at 510 nm. The method employed a Thermo Fischer Scientific Indiko Plus automatic analyzer and a workstation equipped with Indiko software version 5.3; measurements were carried out in kinetic mode. Internal quality control was ensured by analyzing two commercial reference materials in each analysis sequence. External quality and accuracy of the analytical method were assessed by participating in interlaboratory comparison programs, including the College of American Pathologists Forensic Urine Drug Testing (Confirmatory) Survey.
References
- Aranda-Rodriguez, R., Cabecinha, A., Harvie, J., Jin, Z., Marchand, A., Tardif, R., Nong, A., and Haddad, S. (2015). A method for quantification of volatile organic compounds in blood by SPME-GC-MS/MS with broader application: From non-occupational exposure population to exposure studies. Journal of Chromatography B, 992, 76–85.
- CDC (Centers for Disease Control and Prevention) (2011). Bisphenol A and other environmental phenols and Parabens in urine NHANES 2009-2010. Method No. 6301.01. National Center for Environmental Health, Centers for Disease Control and Prevention, Atlanta, GA. Retrieved January 22, 2019.
- Devoy, J., Géhin, A., Müller, S., Melczer, M., Remy, A., Antoine, G., and Sponne, I. (2016). Evaluation of chromium in red blood cells as an indicator of exposure to hexavalent chromium: An in vitro study. Toxicology Letters, 255, 63–70.
- Health Canada (2014). Determination of N-terminal hemoglobin adducts of acrylamide and glycidamide in whole blood by LC/MS/MS. Ontario Food Laboratory, Regions and Programs Bureau, Health Canada, Toronto, ON.
- Health Canada (2017). Analysis of parabens in human urine by UPLC MS MS. BC Food Laboratory. Regulatory Operations and Regions Branch, Health Canada, Vancouver, BC.
- Health Canada (2018). Volatile organic compounds (VOCs) in whole blood by solid-phase microextraction GC-MS/MS. Exposure and Biomonitoring Division, Healthy Environments and Consumer Safety Branch, Health Canada, Ottawa, ON.
- INSPQ (Institut national de santé publique du Québec) (2015a). Analytical method for the determination of acephate and metamidophos in urine by UPLC-MS-MS (E-494), condensed version for CHMS. Laboratoire de toxicologie, Québec, QC.
- INSPQ (Institut national de santé publique du Québec) (2015b). Méthode d'analyse pour doser DCA métabolite du malathion dans l'urine par GC-MS-MS (E-488-B), format condensé pour ECMS. Laboratoire de toxicologie, Québec, QC.
- INSPQ (Institut national de santé publique du Québec) (2015c). Méthode d'analyse pour doser le 3,5,6-trichloro-2-pyridinol dans l'urine par UPLC-MS-MS (E-498-B), format condensé pour ECMS. Laboratoire de toxicologie, Québec, QC.
- INSPQ (Institut national de santé publique du Québec) (2018a). Méthode d'analyse pour doser la cotinine dans l'urine par UPLC-MS-MS méthode robotisée (C-550-G), format condensé pour ECMS. Laboratoire de toxicologie, Québec, QC.
- INSPQ (Institut national de santé publique du Québec) (2018b). Méthode d'analyse pour doser la créatinine dans l'urine par analyseur automatique Indiko Plus (C-601-02), format condensé pour ECMS. Laboratoire de toxicologie, Québec, QC.
- INSPQ (Institut national de santé publique du Québec) (2018c). Méthode d'analyse pour doser la nicotine et ses métabolites et l'anabasine dans l'urine par UPLC-MS-MS (C-569-E), format condensé pour ECMS. Laboratoire de toxicologie, Québec, QC.
- INSPQ (Institut national de santé publique du Québec) (2018d). Méthode d'analyse pour doser le bisphénol A (BPA) et le triclosan dans l'urine par GC-MS-MS (E-475-04), format condensé pour ECMS. Laboratoire de toxicologie, Québec, QC.
- INSPQ (Institut national de santé publique du Québec) (2018e). Méthode d'analyse pour doser le bore urinaire par spectrométrie de masse en tandem à plasma d'argon induit (ICP-MS-MS), Agilent 8800 (M-611-02), format condensé pour ECMS. Laboratoire de toxicologie, Québec, QC.
- INSPQ (Institut national de santé publique du Québec) (2018f). Méthode d'analyse pour doser le chrome hexavalent dans les globules rouges par spectrométrie de masse en tandem à plasma d'argon induit (ICP-MS-MS), Agilent 8800 (M-610-A), format condensé pour ECMS. Laboratoire de toxicologie, Québec, QC.
- INSPQ (Institut national de santé publique du Québec) (2018g). Méthode d'analyse pour doser le ETU total dans l'urine par UPLC-MS-MS (E-499-02), format condensé pour ECMS. Laboratoire de toxicologie, Québec, QC.
- INSPQ (Institut national de santé publique du Québec) (2018h). Méthode d'analyse pour doser les alkylphosphates dans l'urine par GC-MS-MS (E-495-04), format condensé pour ECMS. Laboratoire de toxicologie, Québec, QC.
- INSPQ (Institut national de santé publique du Québec) (2018i). Méthode d'analyse pour doser les composés perfluorés (PFC) dans le sérum / plasma par UPLC-MS-MS (E-501-03), format condensé pour ECMS. Laboratoire de toxicologie, Québec, QC.
- INSPQ (Institut national de santé publique du Québec) (2018j). Méthode d'analyse pour doser les espèces d'arsenic dans l'urine par chromatographie liquide haute pression Waters Acquity en tandem avec la spectrométrie de masse à plasma d'argon induit NexION 350s (HPLC-ICP-MS) (M-612-03), format condensé pour ECMS. Laboratoire de toxicologie, Québec, QC.
- INSPQ (Institut national de santé publique du Québec) (2018k). Méthode d'analyse pour doser les espèces de mercure dans le sang par dilution isotopique en chromatographie gazeuse en tandem avec la spectrométrie de masse à plasma d'argon induit NexION 350s (ID-GC-ICP-MS) (M-613-01), format condensé pour ECMS. Laboratoire de toxicologie, Québec, QC.
- INSPQ (Institut national de santé publique du Québec) (2018l). Méthode d'analyse pour doser les métabolites des phtalates dans l'urine par UPLC-MS-MS (E-490-05), format condensé pour ECMS. Laboratoire de toxicologie, Québec, QC.
- INSPQ (Institut national de santé publique du Québec) (2018m). Méthode d'analyse pour doser les métabolites des pyréthroïdes dans l'urine par GC-MS (E-491-04), format condensé pour ECMS. Laboratoire de toxicologie, Québec, QC.
- INSPQ (Institut national de santé publique du Québec) (2018n). Méthode d'analyse pour doser les métabolites du DINCH et du TOTM dans l'urine par UPLC-MS-MS (E-496-02), format condensé pour ECMS. Laboratoire de toxicologie, Québec, QC.
- INSPQ (Institut national de santé publique du Québec) (2018o). Méthode d'analyse pour doser les métabolites du TXIB et le CHDA dans l'urine par UPLC-MS-MS (E-497-02), format condensé pour ECMS. Laboratoire de toxicologie, Québec, QC.
- INSPQ (Institut national de santé publique du Québec) (2018p). Méthode d'analyse pour doser les métaux et autres éléments dans le sang par spectrométrie de masse à plasma d'argon induit (ICP-MS), DRC II (M-572-10), format condensé pour ECMS. Laboratoire de toxicologie, Québec, QC.
- INSPQ (Institut national de santé publique du Québec) (2018q). Méthode d'analyse pour doser les métaux et autres éléments dans l'urine par spectrométrie de masse à plasma d'argon induit (ICP-MS), DRC II (M-571-09), format condensé pour ECMS. Laboratoire de toxicologie, Québec, QC.
- INSPQ (Institut national de santé publique du Québec) (2018r). Méthode d'analyse pour doser l'ortho-phénylphénol-glucuronide et l'ortho-phénylphénol-sulfate dans l'urine par UPLC-MS-MS (E-503-A), format condensé pour ECMS. Laboratoire de toxicologie, Québec, QC.
6 Statistical data analyses
Descriptive statistics on the concentrations of environmental chemicals in the blood, urine, and hair of Canadians were generated using the Statistical Analysis System software (SAS Institute Inc., version 9.4, 2014) and the SUDAAN® (SUDAAN Release 11.0.1, 2013) statistical software package.
The Canadian Health Measures Survey (CHMS) is a sample survey. This means that the participants represent many other Canadians not included in the survey. In order for the results of the survey to be representative of the entire population, sample weights were generated by Statistics Canada and incorporated into all estimates presented in the data tables. Survey weights were used to take into account the unequal probability of selection into the survey as well as non-response. Further, to account for the complex survey design of the CHMS, the set of bootstrap weights included with the data set was used to estimate the 95% confidence intervals (CIs) for all means, percentiles and detection frequencies (Rao et al., 1992; Rust and Rao, 1996).
Data tables are presented for each chemical measured in cycle 5. When available, data from previous cycles are also provided within the tables. In the first Report on Biomonitoring of Environmental Chemicals in Canada, all results were reported to two decimal places. For subsequent cycles of the CHMS, the reporting protocol changed and the results were reported to two significant digits. For consistency, cycle 1 data were adjusted to two significant digits before generating the descriptive statistics, and data from all cycles are presented to two significant digits. Therefore, the descriptive statistics presented for cycle 1 may differ from those presented in the first report. The differences are not significant and the values presented in the first report are still considered to be accurate.
The data tables include the sample size (n); the percentage of the population with concentrations at or above the limit of detection (LOD), termed detection frequency; the geometric mean (GM); and the 10th, 50th, 90th, and 95th percentiles with associated 95% CIs. For each chemical, results are presented for the total population as well as by age group and sex. Measurements that fell below the LOD for the laboratory analytical method were assigned a value equal to half the LOD. If the proportion of results below the LOD was greater than 40%, GMs were not calculated. Percentile estimates that are less than the LOD are reported as <LOD. LOD values for each chemical are provided alongside their respective data tables and in Appendix A. Conversion factors to assist in the comparison of data from other studies that report different units are provided in Appendix B.
Chemicals measured in either whole blood or plasma are presented as weight of chemical per volume of whole blood or plasma (µg chemical/L blood or plasma). Data for hemoglobin adducts are presented as the amount of hemoglobin adduct per weight of hemoglobin (pmol adduct/g hemoglobin). Chromium (VI) measures in red blood cells are presented as weight of chromium per volume of red blood cells (µg/L red blood cells).
For urine measurements, concentrations are presented as weight of chemical per volume of urine (µg chemical/L urine) and adjusted for urinary creatinine (µg chemical/g creatinine). Urinary creatinine is a chemical by-product generated from muscle metabolism; it is frequently used to adjust for urine concentration (or dilution) in spot urine samples because its production and excretion are relatively constant over 24 hours owing to homeostatic controls (Barr et al., 2005; Boeniger et al., 1993; Pearson et al., 2009). If the chemical measured behaves similarly to creatinine in the kidney, it will be filtered at the same rate; thus, expressing the chemical per gram of creatinine helps adjust for the effect of urinary dilution as well as some differences in renal function and lean body mass (Barr et al., 2005; CDC, 2009; Pearson et al., 2009). Creatinine is primarily excreted by glomerular filtration; therefore, creatinine adjustment may not be appropriate for compounds that are excreted primarily by tubular secretion in the kidney (Barr et al., 2005; Teass et al., 2003). In addition, creatinine excretion can vary based on age, sex, and ethnicity; therefore, it may not be appropriate to compare creatinine-adjusted concentrations among different demographic groups (e.g., children and adults) (Barr et al., 2005). Where urinary creatinine values were missing or <LOD, the estimate of that participant's creatinine-adjusted chemical was not calculated and was also listed as missing.
Descriptive statistics are available for creatinine (mg/dL) (Appendix C). These include n; detection frequency; GM; the 10th, 50th, 90th, and 95th percentiles; and associated 95% CIs for the total population as well as by age group and sex. Measurements that fell below the LOD for the laboratory analytical method were assigned a value equal to half the LOD.
Specific gravity was also measured in all urine samples immediately following sample collection at the mobile examination centre. Urinary specific gravity is the ratio of densities between urine and pure water, and can be used to adjust for variations in urine output, similar to urinary creatinine adjustment. Urinary specific gravity adjustment has not been presented for any of the chemicals; however, specific gravity data are available upon request by contacting Statistics Canada at infostats@canada.ca should researchers wish to perform this adjustment for their own data analyses.
Under the Statistics Act, Statistics Canada is required to ensure participant confidentiality. Therefore, estimates based on a small number of participants are suppressed. Following suppression rules for the CHMS, any estimate based on fewer than 10 participants is suppressed in the data tables. To avoid suppression, estimates at the 95th percentile require at least 200 participants; estimates at the 10th and 90th percentiles require at least 100 participants; estimates at the 50th percentile require at least 20 participants; and estimates of the GM require at least 10 participants.
Estimates from a sample survey will inevitably include sampling errors. Measuring the possible scope of sampling errors is based on the standard error of the estimates drawn from the survey results. To get a better indication of the size of the standard error, it is often more useful to express the standard error in terms of the estimate being measured. The resulting measure, called the coefficient of variation (CV), is obtained by dividing the standard error of the estimate by the estimate itself; it is expressed as a percentage of the estimate. This report employs the following Statistics Canada guidelines for releasing estimates based on their CVs:
- When a CV is between 16.6% and 33.3%, an estimate can be considered for general unrestricted release, but is accompanied by a warning that cautions subsequent users of the high sampling variability associated with the estimate. These estimates are identified by the superscript letter E.
- When a CV is greater than 33.3%, Statistics Canada recommends not releasing the estimate because conclusions based on these data will be unreliable and most likely invalid. These estimates will not be published and will instead be replaced by the letter F.
Further details on the sample weights and data analysis are available in the Canadian Health Measures Survey (CHMS) Data User Guide: Cycle 5 (Statistics Canada, 2019).
References
- Barr, D.B., Wilder, L.C., Caudill, S.P., Gonzalez, A.J., Needham, L.L., and Pirkle, J.L. (2005). Urinary creatinine concentrations in the U.S. population: Implications for urinary biologic monitoring measurements. Environmental Health Perspectives, 113(2), 192–200.
- Boeniger, M.F., Lowry, L.K., and Rosenberg, J. (1993). Interpretation of urine results used to assess chemical exposure with emphasis on creatinine adjustments: A review. American Industrial Hygiene Association Journal, 54(10), 615–627.
- CDC (Centers for Disease Control and Prevention) (2009). Fourth national report on human exposure to environmental chemicals. Department of Health and Human Services, Atlanta, GA. Retrieved June 28, 2019.
- Pearson, M., Lu, C., Schmotzer, B., Waller, L., and Riederer, A. (2009). Evaluation of physiological measures for correcting variation in urinary output: Implications for assessing environmental chemical exposure in children. Journal of Exposure Science and Environmental Epidemiology, 19(3), 336–342.
- Rao, J., Wu, C., and Yue, K. (1992). Some recent work on resampling methods for complex surveys. Survey Methodology, 18(2), 209–217.
- Rust, K.F., and Rao, J.N.K. (1996). Variance estimation for complex surveys using replication techniques. Statistical Methods in Medical Research, 5(3), 283–310.
- Statistics Canada (2019). Canadian Health Measures Survey (CHMS) Data User Guide: Cycle 5. Ottawa, ON. Available upon request (infostats@canada.ca).
- Teass, A., Biagini, R., DeBord, G., and DeLon Hull, R. (2003). Application of biological monitoring methods. NIOSH Manual of Analytical Methods, NIOSH Publication Number 2003–154(3).
7 Considerations for interpreting the biomonitoring data
The Canadian Health Measures Survey (CHMS) was designed to provide estimates of environmental chemical concentrations in blood or urine for the Canadian population as a whole. The first cycle of the survey covered approximately 96% of the Canadian population aged 6–79 years. The subsequent cycles included children as young as three years of age and covered approximately 96% to 97% of the Canadian population aged 3–79 years of age. For cycle 5, hair measures were added for a subset of the adult population aged 20–59 years. The survey was not designed to permit breakdown of data by region, province, or collection site, although some analysis is possible if data from more than one cycle are combined (see Instructions for Combining Multiple Cycles of Canadian Health Measures Survey [CHMS] Data [Statistics Canada, 2015]). In addition, the CHMS design did not target specific exposure scenarios; consequently, it did not select or exclude participants on the basis of their potential for low or high exposures to environmental chemicals.
Biomonitoring can estimate how much of a chemical is present in a person, but it cannot say what health effects, if any, may result from that exposure. The ability to measure environmental chemicals at very low concentrations has advanced in recent years. However, the presence alone of a chemical in a person's body does not necessarily mean that it will cause a health effect. Factors such as the dose, the toxicity of the chemical, and the duration and timing of exposure are important to determine whether potential adverse health effects may occur. For chemicals such as lead or mercury, research studies have provided a good understanding of the health risks associated with different concentrations in blood. However, for many chemicals, further research is needed to understand the potential health effects, if any, associated with different blood, urine, or hair concentrations. Furthermore, small amounts of certain chemicals, such as selenium, are essential for the maintenance of good health and would be expected to be present in the body. In addition, the way in which a chemical will act in the body will differ among individuals and cannot be predicted with certainty. Certain populations (children, pregnant women, the elderly, or immunocompromized people) may be more susceptible to the effects of exposure.
The absence of a chemical does not necessarily mean a person has not been exposed. It may be that the technology is not capable of detecting such a small amount, or that the exposure occurred at an earlier point in time, allowing for the chemical to be eliminated from the person's body before the measurement took place.
Biomonitoring cannot tell us the source or route of the exposure. The amount of chemical measured indicates the total amount that has entered the body through all routes of exposure (ingestion, inhalation, and skin contact) and from all sources (air, water, soil, food, and consumer products). The detection of the chemical may be the result of exposure to a single source or multiple sources. In addition, in most cases, biomonitoring cannot distinguish between natural and anthropogenic sources. Many chemicals (lead, mercury, cadmium, and arsenic) occur naturally in the environment and are also present in human-made products.
While most metals are measured as the parent compounds, many other chemicals are measured as metabolites. For many chemicals, parent compounds may be broken down (i.e., metabolized) in the body into one or more metabolites. For example, the pyrethroid pesticide deltamethrin is broken down into several metabolites. Some metabolites are specific to one parent compound, whereas others are common to several parent compounds. As well, several metabolites found in urine are also found in the environment as a result of other processes (e.g., dialkyl phosphate metabolites). Their presence in urine does not necessarily mean that an exposure to the parent chemical has occurred; rather, exposure could be to the metabolite itself in media such as food, water, or air.
Factors that contribute to the concentrations of chemicals measured in blood, urine and hair include the quantity entering the body through all routes of exposure, absorption rates, distribution to various tissues in the body, metabolism, and excretion of the chemical and/or its metabolites from the body. These processes, also called toxicokinetics, depend on both the characteristics of the chemical, including its solubility in fat (or lipophilicity), its pH, its particle size, and the characteristics of the individual being exposed, such as age, diet, health status, and ethnicity. For these reasons, the way in which a chemical will act in the body will differ among individuals and cannot be predicted with certainty.
The CHMS biomonitoring data currently available include temporal data for substances measured in cycle 1 (2007–2009), cycle 2 (2009–2011), cycle 3 (2012–2013), cycle 4 (2014–2015), and cycle 5 (2016–2017). Results from future cycles can be compared with the baseline data from the CHMS in order to examine trends in Canadians' exposures to selected environmental chemicals. It is important to note that some sampling and analytical modifications between cycles may have contributed some variation in results for those substances measured in multiple cycles. The limits of detection (LODs) for certain analytical methods have changed from cycle to cycle (Appendix A). Although the LOD values did not change by a large margin, this difference should be noted when comparing data from multiple cycles. In addition, the urine collection protocol and guidelines were changed in cycle 2, and this may have resulted in a shift in creatinine levels when cycle 1 data are compared with those from subsequent cycles. This, in turn, could affect creatinine-adjusted levels of some chemicals.
Urinary creatinine concentrations can also be affected by variables such as age, sex, and ethnicity, resulting in differences among demographic groups within a single cycle (Mage et al., 2004). In particular, creatinine excretion per unit of body weight increases substantially with increasing age in children (Aylward et al., 2011; Remer et al., 2002). As a result, it is acceptable to compare creatinine-adjusted concentrations among similar demographic groups (e.g., children with children, adults with adults, males with males) but not among two different demographic groups (e.g., children with adults, males with females) (Barr et al., 2005).
More in-depth statistical analyses of the CHMS biomonitoring data — including time trends, exploring relationships among environmental chemicals, other physical measures, and self-reported information — are being published by researchers in scientific literature. A bibliography of publications using CHMS data is available. CHMS data are available to scientists through Statistics Canada's Research Data Centres Program and are a resource for additional scientific analyses. Further information about the CHMS can be obtained by contacting Statistics Canada at infostats@canada.ca.
References
- Aylward, L.L., Lorber, M., and Hays, S.M. (2011). Urinary DEHP metabolites and fasting time in NHANES. Journal of Exposure Science and Environmental Epidemiology, 21(6), 615–624.
- Barr, D.B., Wilder, L.C., Caudill, S.P., Gonzalez, A.J., Needham, L.L., and Pirkle, J.L. (2005). Urinary creatinine concentrations in the U.S. population: Implications for urinary biologic monitoring measurements. Environmental Health Perspectives, 113(2), 192–200.
- Mage, D.T., Allen, R.H., Gondy, G., Smith, W., Barr, D.B., and Needham, L.L. (2004). Estimating pesticide dose from urinary pesticide concentration data by creatinine correction in the Third National Health and Nutrition Examination Survey (NHANES-III). Journal of Exposure Analysis and Environmental Epidemiology, 14(6), 457–465.
- Remer, T., Neubert, A., and Maser-Gluth, C. (2002). Anthropometry-based reference values for 24-h urinary creatinine excretion during growth and their use in endocrine and nutritional research. American Journal of Clinical Nutrition, 75(3), 561–569.
- Statistics Canada (2015). Instructions for Combining Multiple Cycles of Canadian Health Measures Survey (CHMS) Data. Ottawa, ON. Available upon request (infostats@canada.ca).
Page details
- Date modified: